Disembodied Mind: Cortical Changes Following Brainstem Injury in Patients with Locked-in Syndrome
Abstract
Locked-in syndrome (LIS) following ventral brainstem damage is the most severe form of motor disability. Patients are completely entrapped in an unresponsive body despite consciousness is preserved. Although the main feature of LIS is this extreme motor impairment, minor non-motor dysfunctions such as motor imagery defects and impaired emotional recognition have been reported suggesting an alteration of embodied cognition, defined as the effects that the body and its performances may have on cognitive domains. We investigated the presence of structural cortical changes in LIS, which may account for the reported cognitive dysfunctions. For this aim, magnetic resonance imaging scans were acquired in 11 patients with LIS (6 males and 5 females; mean age: 52.3±5.2SD years; mean time interval from injury to evaluation: 9±1.2SD months) and 44 healthy control subjects matching patients for age, sex and education. Freesurfer software was used to process data and to estimate cortical volumes in LIS patients as compared to healthy subjects. Results showed a selective cortical volume loss in patients involving the superior frontal gyrus, the pars opercularis and the insular cortex in the left hemisphere, and the superior and medium frontal gyrus, the pars opercularis, the insular cortex, and the superior parietal lobule in the right hemisphere. As these structures are typically associated with the mirror neuron system, which represents the neural substrate for embodied simulation processes, our results provide neuroanatomical support for potential disembodiment in LIS.
INTRODUCTION
Locked-in syndrome (LIS) represents the most severe form of motor disability after brain injury [1]. It is the consequence of ventral brainstem damage as a result of vascular or traumatic lesions disconnecting corticospinal and corticobulbar tracts bilaterally (Fig. 1). Patients with LIS are fully conscious but completely paralyzed with the exception of vertical eye movements and blinking. They also show anarthria and lower cranial nerve paralysis and learn to interact with others through an eye-coded communication system. As supratentorial structures and cortex are considered traditionally preserved in these patients, they have been long regarded as cognitively intact. Recent studies, however, have described some cognitive and behavioral dysfunctions, including motor imagery defects, impaired recognition of negative facial expressions and pathologic laughter and crying [2-5]. Moreover, these dysfunctions are associated with a functional impairment of cortical neuronal synchronization mechanisms in the resting state condition [6]. All together these observations suggest that patients with LIS may progressively develop cortical changes, which may be responsible for unexpected behavioral disorders. Actually, it is reasonable to expect that patients with LIS show a certain degree of selective cortical atrophy due to the disconnection between the cortex and the spinal cord. This assumption is endorsed by data on other categories of patients, such as patients with spinal cord injury, in whom changes in brain motor activation patterns, after the initial spine injury, have been described [7, 8]. Similarly, the temporary immobilization of a limb in healthy subjects has been reported to induce immobilization-related plasticity phenomena in the motor imagery circuitry, thus endorsing the idea that limb non-use may lead to corticomotor depression and atrophy [9]. To date, alterations in gray matter volume have never been investigated in patients with LIS. The aim of this study is to investigate the presence of structural cortical changes following brainstem damage in LIS.
METHODS
Participants
All patients with LIS consecutively admitted to the Post-Coma Rehabilitation Care Unit of the San Raffaele Hospital, Cassino, Italy, within a two-year period were included in the study. Inclusion criteria were diagnosis of LIS at admission as a consequence of ventral brainstem damage of traumatic or vascular origin, age ≥18 years, and informed consent by the proxy or surrogate to participate in this study. Exclusion criteria were the instability of general clinical conditions after two weeks from admission, the presence of additional cortical or subcortical lesions as shown by Magnetic Resonance (MR) imaging, the observation of eye movement disorders as a further impediment to communication and a history of psychiatric or cognitive dysfunctions before the brainstem injury. The assessment resulting in the diagnosis of LIS was carried out within the first week from admission, by expert neurologists (FP and SS) previously trained to assess such patients. Screened patients were classified according to the Bauer’s classification, which stratifies patients into three categories based upon the degree of residual ability to communicate: the classic or pure form, characterized by the complete loss of any motor output with the exception of vertical eye movements and blinking; the incomplete form, characterized by the presence of some voluntary motion other than eye movements; and the total form, characterized by the complete loss of any motor output, including eye movements [1, 10, 11].
Healthy volunteers matching patients for age, sex and education, free from neurological or psychiatric disorders and with normal MR imaging, were also included in the study.
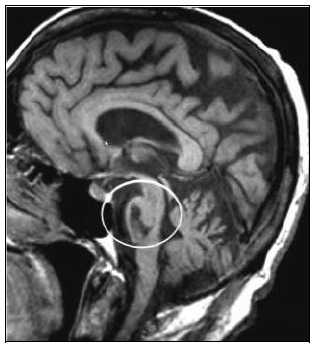
Ventral brainstem lesion in locked-in syndrome.
Procedures
MRI Acquisition
A 1.5 Tesla MRI scanner (Espree, Siemens AG, Erlangen) with a standard 8-channel birdcage head coil was used for MRI data acquisition. For each subject and each time point, two images were acquired. The first was a high-resolution T1-weighted image (2 times), acquired using a Magnetization Prepared Rapid Acquisition Gradient Echo (MPRAGE) sequence (repetition time 1590ms; echo time 2.4ms; flip angle 0°; matrix size 192 × 192; number of slices 160; voxel size 1 × 1 × 1mm3). The second was a T2-weighted image using a Fluid Attenuated Inversion Recovery (FLAIR) sequence (repetition time 9000ms; echo time 88ms; flip angle 0°; matrix size 384 × 512; number of slices 44).
All brain MRI scans were reviewed independently by two experienced neuroradiologists (CCQ and CAM) in order to rule out additional cortical or subcortical structural abnormalities.
Volumetric Analysis
Cortical reconstruction and volumetric segmentation was performed through the Freesurfer image analysis suite, which is documented and freely available for download online at (surfer.nmr.mgh.harvard.edu/). Freesurfer volumetric analysis has been widely used across past studies addressing various issues [12-15]. The technical details of Freesurfer-based procedures are described in prior publications [16-27]. Briefly, this processing includes motion correction and averaging of multiple volumetric T1 weighted images [28], removal of non-brain tissue using a hybrid watershed/surface deformation procedure [27], automated Talairach transformation, segmentation of the subcortical white matter and intensity normalization of deep gray matter volumetric structures (including hippocampus, amygdala, caudate, putamen and ventricles) [20, 21-29], tessellation of the gray matter-white matter boundary, automated topology correction [19, 30] and surface deformation following intensity gradients to optimally place the gray/white and gray/cerebrospinal fluid borders at the location where the greatest shift in intensity defines the transition to the other tissue class [16-18]. Once the cortical models are completed, a number of deformable procedures can be performed for further data processing and analysis. These include surface inflation [22], registration to a spherical atlas which utilizes individual cortical folding patterns to match cortical geometry across subjects [23], parcellation of the cerebral cortex into units based on gyral and sulcal structure [24], and creation of a variety of surface based data including maps of curvature and sulcal depth. This method uses both intensity and continuity information from the entire three dimensional MR volume in segmentation and deformation procedures to produce representations of cortical thickness, calculated as the closest distance from the gray/white boundary to the gray/CSF boundary at each vertex on the tessellated surface [18]. In this study, segmented and skull stripped data of all participants were visually inspected and inaccuracies, if any, were manually corrected by expert neuroradiologists (CCQ, CAM) to avoid misclassification of tissue type, then edited again. Finally, maps were smoothed by a Gaussian Kernel of 10mm Full Width at Half Maximum (FWHM). For each participant, mean cortical volume values of 34 brain regions, according to Desikan parcellation [31], were calculated for both hemispheres as the average of two values, the minimal distance from gray/white matter boundary and pial surface and vice versa. Each subject’s brain was morphed and registered to an average spherical surface that finely aligns sulci and gyri across them. Then cortical volume values were mapped on this average inflated surface, thus avoiding interference of cortical folding on the visualization.
Statistical maps were generated using FreeSurfer’s Query, Design, Estimate, Contrast (QDEC) interface. For each hemisphere a general linear model (GLM) of the effect of age on cortical volumes was evaluated at each vertex for male and female groups. Spatial smoothing with an isotropic kernel (FWHM =10 mm) was applied. Data were deemed as statistically significant if p<0.01. False Discovery Rate (FDR) was corrected and tables of cluster size and location were generated.
RESULTS
Study Participants
Out of 13 patients who were admitted within the study period, 11 patients fulfilled the criteria to be included in the study (6 males and 5 females; mean age: 52.3±5.2SD years, range 40–66; mean education: 12±4.1SD years, range 5-17; mean time interval from injury to evaluation: 9±1.2SD months). Two patients were excluded (1 male and 1 female; mean age: 48.5±19.0SD years) as showing ocular movement disorders (n = 1) or additional cortical lesions as a result of a previous vascular injury (n = 1). All included patients showed a pure LIS. In addition, forty-four right-handed healthy subjects, matching patients for age, sex, and education, were included as controls.
Cortical Volume Vertex Comparison Between Groups
After computing the whole brain data and exploring brain regions in which cortical volume vertex varied between groups, lower cortical volumes in the locked-in group were detected in the following structures (data are presented in descending order for each hemisphere): in the left hemisphere, insula (log(10)t = -3.2873 size = 106.31 mm2 x = -33.8 y = 5.5 z = -13.8), superior frontal gyrus (log(10)t = -2.9907 size= 172.08 mm2 x = -10.0 y = 46.8 z = 40.4), pars opercularis (log(10)t = -2.9773 size = 811.70 mm2 x= -48.4 y = 8.3 z = 1.1); in the right hemisphere, insula (log(10)t = -3.3260 size = 131.29 mm2 x = 38.2 y = -14.1 z = 7.4), superior parietal lobule (log(10)t = -3.2355 size = 145.46 mm2 x = 15.4 y = -72.9 z = 41.0), rostral middle frontal region (log(10)t = -2.8092 size = 130.57 mm2 x = 35.7 y = 32.9 z = 30.3), cuneus (log(10)t = -2.6725 size = 116.00 mm2 x = 5.2 y = -80.4 z = 26.2), post-central gyrus (log(10)t = -2.6316 size = 108.72 mm2 x = 57.3 y = -9.7 z = 30.3), pars opercularis (log(10)t = -2.5917 size = 140.62mm2 x= 49.8 y = 11.9 z = 2.9) and precuneus (log(10)t = -2.5099 size = 137.23mm2 x = 21.6 y = -68.3 z = 24.1). Cortical alterations are shown in Figs. (2, 3). More detailed data from the volumetric analysis are reported in the Table 1.
Detailed data of volumetric analysis for left and right hemisphere respectively.
![]() |
DISCUSSION
In the present study we investigated possible alterations in gray matter volume following brainstem damage in LIS. Results showed a selective cortical volume loss in LIS patients as compared to healthy controls. In the left hemisphere, cortical loss mainly involved the superior frontal gyrus, the pars opercularis and the insular cortex, while in the right hemisphere loss was mainly detected within the superior and medium frontal gyrus, pars opercularis, insular cortex, superior parietal lobule, cuneus, and precuneus. Lateral frontal and parietal cortices, including the parietal lobule, posterior inferior frontal gyrus and adjacent ventral premotor cortex, have typically been associated with the classical mirror neuron system, which represents the neural substrate for imitation and action understanding [32]. Later behavioral and neurofunctional studies extended the mirror neuron system areas beyond those belonging to the classical fronto-parietal system [33, 34] and specified a wider range of related cognitive processes, other than action observation and imitation. Nowadays this broader system is at the heart of the concept of embodied simulation which refers to our ability to recognize actions from other people by simulating in our own minds the same experiences [35]. Different views of simulation are presented in the literature, but almost all share the idea that the same motor representations are involved not only in imitation or action observation but also in other action-related phenomena such as motor imagery [36]. In recent years, it has been demonstrated that patients with LIS are affected by motor imagery deficits, as demonstrated by their impaired performance on mental rotation of hand images in the presence of a spared ability to mentally rotate non-corporeal images such as three-dimensional geometrical figures [2, 3]. It has been suggested that defective action simulation processes in LIS must be ascribed to the functional interruption of the parieto-cerebellar circuit specifically involved in movement prediction [37]. The findings of the present study allow us to further clarify the mechanisms underlying motor imagery deficits in LIS patients and to hypothesize that the reported gray matter volume alterations in fronto-parietal cortical areas may be responsible for an alteration of embodied cognition. The cortical loss which was found in medial occipital (cuneus) and parietal areas (precuneus) further support this interpretation, as previous neuroimaing evidence from healthy individuals and from patients with anorexia nervosa showed the involvement of these areas in motor imagery and in alterations of self-body representation [38, 39]. Recently, it has also been demonstrated that LIS patients are significantly less accurate than healthy controls in recognizing negative emotional facial expressions and tend to confound between different negative emotions [4]. On the contrary, they can accurately identify non-negative emotional expressions (happiness and surprise) [4]. Since embodied simulation theories posit that reenactment of sensory and motor components related to emotional experience contributes to the attribution of emotions to others [40], it has been suggested that impaired voluntary mimicry in LIS could affect conscious recognition of facial expressions (without hampering adequate emotional responses to complex scenes) [4].
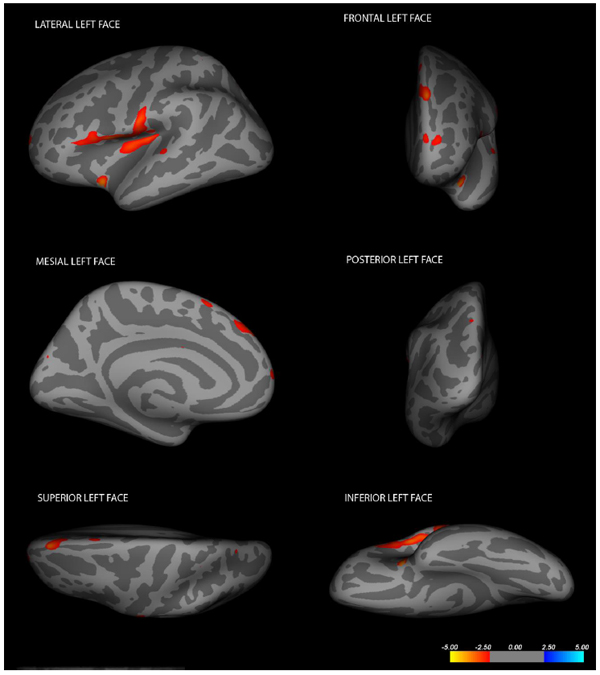
Cortical changes in LIS patients in the left hemisphere (Changes have been estimated by comparing LIS patients and healthy controls).
In this respect, a more recent study examined activation patterns associated with the execution and/or observation of expressions of emotions and found that, in addition to classical fronto-parietal mirror regions, simulation of emotional states also involved the amygdala, the cingulate and the insula [41]. The present results, showing a cortical loss within the left and right insula, are consistent with evidence on “emotional simulative brain areas” and provide further support for the idea that a lesion of the ventral pons, interrupting efferent pathways, as in LIS patients, also interferes with functional connections linking the frontal, parietal, and limbic cortex with the cerebellum via pontine nuclei, leading to a severe defect of embodied simulation processes [4, 42, 43]. It may be that the functional interruption of parieto-cerebellar connections abolishes forward mechanisms predicting the consequences of movements, accounting for motor imagery defects and for the selective impairment of explicit recognition of emotional facial expressions in LIS. The strength of our study includes the case-control design, the large sample size and the robustness of the adopted statistical method. A limitation is represented by the absence of a concurrent cognitive assessment in the investigated patients. Future studies addressing this issue are needed in order to identify both cortical changes and cognitive dysfunctions in patients with LIS and to determine correlations between each other.
CONCLUSION
Despite the main feature of LIS syndrome remains the extreme motor impairment, several minor cognitive dysfunctions have been described. These dysfunctions may be the result of alterations in embodied cognition, defined as the effect that the body and its performances can have on cognitive domains. This allows us to hypothesize the presence of inverse plasticity phenomena on embodiment related areas in patients with LIS. In fact, a LIS patient cannot “bring into play” the mayor embodied functions when interacting with the environment. In this way, the preserved working of cortical embodiment-related areas may result in a confounding source of noise in everyday life. Therefore, the combination of motor and non-motor symptoms in LIS may be the consequence of the ventral pontine damage coupled with an adaptive inhibition of most embodiment-related cortical areas.
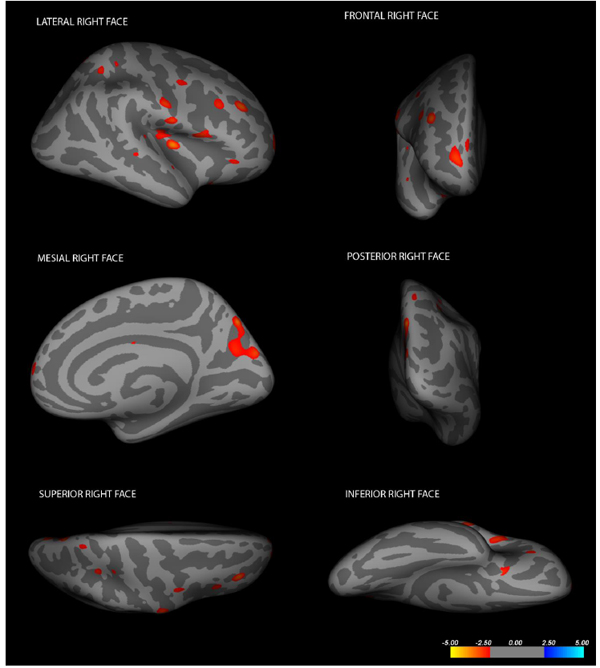
Cortical changes in LIS patients in the right hemisphere (Changes have been estimated by comparing LIS patients and healthy controls).
CONFLICT OF INTEREST
The authors confirm that this article content has no conflict of interest.
ACKNOWLEDGEMENTS
Decleared none.