All published articles of this journal are available on ScienceDirect.
Neurovascular Compression in Arterial Hypertension: Correlation of Clinical Data to 3D-Visualizations of MRI-Findings
Abstract
Aims:
In this study, we attempted to identify clinical parameters predicting the absence or presence of Neurovascular Compression (NVC) at the Ventrolateral Medulla (VLM) in arterial hypertension (HTN) in MRI findings.
Background:
Cardiovascular and pulmonary afferences are transmitted through the left vagus and glossopharyngeal nerve to the brain stem and vasoactive centers. Evidence supports the association between HTN and NVC at the left VLM. Several independent studies indicate a reduction of HTN after Microvascular Decompression (MVD) of the left. Several independent studies indicate a reduction of HTN after Microvascular Decompression (MVD) of the left VLM. Image processing of MRI provides comprehensible detection of NVC. HTN affects hemodynamic parameters and organs.
Objective:
This study analyzes and correlates clinical data and MRI findings in patients with and without NVC at the VLM in treatment resistant HTN to obtain possible selection criteria for neurogenic hypertension.
Methods:
In 44 patients with treatment resistant HTN, we compared MRI findings of neurovascular imaging to demographic, clinical and lifestyle data, office and 24-hour ambulatory Blood Pressure (BP), and cardiovascular imaging and parameters.
Results:
Twenty-nine (66%) patients had evidence of NVC at the VLM in MRI. Sixteen patients (36%) had unilateral NVC on the left side, 7 (16%) unilateral right and 6 (14%) bilateral NVC. Fifteen (34%) had no evidence of NVC at the VLM. Patients with left sided NVC were significantly younger, than those without NVC (p=0.034). They showed a statistically significant variance in daytime (p=0.020) and nighttime diastolic BP (p<0.001) as the mean arterial pressure (p=0.020). Other measured parameters did not show significant differences between the two groups.
Conclusion:
We suggest to examine young adults with treatment resistant HTN for the presence of NVC at VLM, before signs of permanent organ damage appear. Clinical and hemodynamic parameters did not emerge as selection criteria to predict NVC. MVD as a surgical treatment of NVC in HTN is not routine yet as a surgical treatment of NVC in HTN is not routine yet. Detection of NVC by imaging and image processing remains the only criteria to suggest MVD, which should be indicated on an individual decision.
1. INTRODUCTION
NVC describes a pathological contact between the Root Entry/Exit Zone (REZ) of a cranial nerve and a vessel at the brain stem, resulting in a hyperactive dysfunction. A possible etiologic association between NVC at the left VLM and arterial HTN was noticed by JANNETTA et al. [1]. A significant reduction of arterial HTN was observed after microvascular decompression (MVD) [1-3]. A microanatomical study classified the NVC at the VLM in HTN [4, 5]. Animal models have defined the role of the sympathoexcitatory neurons of the rostral VLM and the inhibitory neurons of the caudal VLM in blood pressure regulation [4]. It seems that NVC at the VLM leads to a permanent irritation and activation of the C1 neurons located at the VLM. A possible explanation for the left NVC in essential HTN is the additional compression of the REZ of the ninth and 10th Cranial Nerves (CN IX-X). The major part of the afferent inputs from the myocardial receptors of the left ventricle and atrium to the nucleus tractus solitarii are conducted by the low-myelinated cardiac c-fibers of the left vagus nerve [4]. Several imaging studies supported the evidence of NVC [6-10] opposed by other studies, which could not find a significant association [11-17]. JANNETTA’s surgical results were verified in multiple independent short and long term studies [2, 3, 6]. Elevated sympathetic nerve activity was observed in hypertensive patients with NVC at VLM in magnetic resonance imaging (MRI) [18, 19].
Physical parameters characterizing body size, such as Body Mass Index (BMI), are associated with an elevated BP. HTN itself results in target organ damage like myocardial hypertrophy and vascular changes, which also result in abnormal parameters detected by physical examination and cardiovascular imaging. Elevated BP is present in approximately 80% of patients with ischemic stroke [20, 21]. Furthermore, BP variability (BPV) is an independent cardiovascular risk factor in hypertensive patients [22].
It seems that NVC of the left VLM is correlated to HTN, but only a few studies on the cause and the treatment, either clinical [1, 2, 23, 24] or experimental [1, 25-28], have been reported in the literature despite the possibility that such a neurogenic mechanism might be implicated in a relatively large number of patients [29], so this hypothesis of neurogenic hypertension still remains an object of debate.
The concept of vascular compression of the VLM as a mediator of increased BP via sympathetic stimulation is intriguing and may prove relevant in various clinical scenarios associated with elevated BP and its consequences, including stroke [30]. Until now, there exists no study correlating 3D- visualizations of MRI for detection of NVC at the VLM to hemodynamic and clinical parameters of patients with HTN. In this study, we attempted to identify clinical parameters predicting the absence or presence of NVC in HTN.
The study protocol with the number: 2633 was approved by the local ethics committee and performed according to the Declaration of Helsinki and the guidelines for “Good Clinical Practice” (GCP). Before enrollment, each patient provided written informed consent prior to inclusion in this observational study. The main results with respect to the outcome, BP and sympathetic activity have been previously published [18].
2. MATERIALS AND METHODS
2.1. Clinical Data
The study cohort consisted of 44 patients (8 women and 36 men, age range 32-70 years, mean 57.5 years) with treatment resistant HTN, defined as office BP ≥140/90 mmHg (mean of two measurements), while treated with at least 3 antihypertensive drugs, one of which was a diuretic [31]. True treatment resistant HTN was confirmed by 24-hour ambulatory BP measurements (average 24-hour BP ≥ 130/80 mmHg). All patients were consecutively enrolled and referred to our University outpatient service to rule out secondary causes of HTN and discuss with the patient alternative interventional therapies.
In this prospective observational study, the following clinical parameters were obtained: age, gender, height, weight, body surface (BSA), BMI, family history of HTN, known duration of HTN (months), and duration of medical therapy. Furthermore, with the aid of questionnaires the following parameters were assessed: alcohol consumption (g/week), sporting activity (hours/week) and consumption of nicotine (pack years).
The patients were on a typical Bavarian diet without any restrictions, including salt intake. Otherwise, we would have needed a metabolic ward, which requires a completely different setting.
2.2. Blood Pressure Measurements
Office systolic and diastolic BP as well as heart rate (HR) were captured according to European Society of Hypertension/European Society of Cardiology (ESH/ESC) guideline recommendations. Additionally, we measured the casual systolic and diastolic BP (in mmHg) and the HR (heartbeats/minute) 3 minutes after standing up. Ambulatory 24-hour BP measurements provided averages of 24-hour systolic and diastolic BP, daytime and night-time diastolic and systolic BP, 24 h ambulatory HR and 24 h ambulatory mean arterial pressure. Daytime measurements were taken every 15 minutes and nighttime measurements every 30 minutes. As quality criteria, at least 50 measurements have been successfully completed over a 24-hour period in order to be entered into the database.
2.3. Cardiovascular Parameters
In all patients, a two-dimensional (2-D) guided amplitude mode (A-Mode) echocardiography was conducted to assess left ventricular structure and function. Septal wall thickness, posterior wall thickness and left ventricular mass were calculated, as previously recommended [32].
For an accurate and precise noninvasive determination of left ventricular mass and volume, cardiac MRI was applied [33, 34]. The prognostic significance of left ventricular function was well established. All subjects were imaged on a Siemens Symphony and Sonata MRI (Siemens AG, Erlangen, Germany). Cine images were acquired in 6 equally spaced short-axis locations from apex to base and 3 long-axis locations orthogonal to the short axis and orientated at 60° increments around the left ventricular central axis [34].
A 12-lead electrocardiogram (ECG) was recorded at rest in supine position and the electrocardiographic criteria of left ventricular hypertrophy (LVH) were analyzed: Sokolow-Lyon-Voltage-Index [35] and Cornell voltage duration product (Cornell) [36], heart rate and QT-time (in seconds) were obtained. To measure central systolic pressure and central pulse pressure, radial arterial wave form was recorded by the Sphygmocor System (Atcor-CardieX, New South Wales, Australia) and corresponding central aortic wave form were then automatically generated through a validated transfer function. In addition, pulse wave velocity was measured as a marker of arterial stiffness.
2.4. Imaging Procedures and Image Processing of NVC
Imaging was performed on a 3 Tesla MR System (Magnetom Trio, Siemens AG, Erlangen, Germany). High-resolution Constructive Interference in Steady State (CISS) (repetition time (TR) 7.48 ms, Time of Echo (TE) 3.23 ms, flip angle 45°, acquisition time 8:23 min:sec, matrix 512 x 512, number of slices 144, voxel size 0.4) and a 3D-TOF Angiogram (TR 21 ms, TE 3.77 ms, flip angle 18°, acquisition time 15:51 min:sec, matrix 512 x 512, number of slices 144, voxel size 0.4) were acquired. Image processing was applied to depict neurovascular relationships according to a specific protocol [37-39].
With this technique, we produced 3D representations of the brain stem and cranial nerves together with the vessels at the surface of the brain stem in each individual [38]. The relationships between cranial nerves and vessels, especially at the VLM were analyzed regarding the existence of NVC by two independent expert observers. The location and type of NVC [4] were determined (Fig. 1). The presence or absence of NVC in the 3D representations divided the patients into two groups. The obtained clinical and hemodynamic parameters were correlated between the two groups.
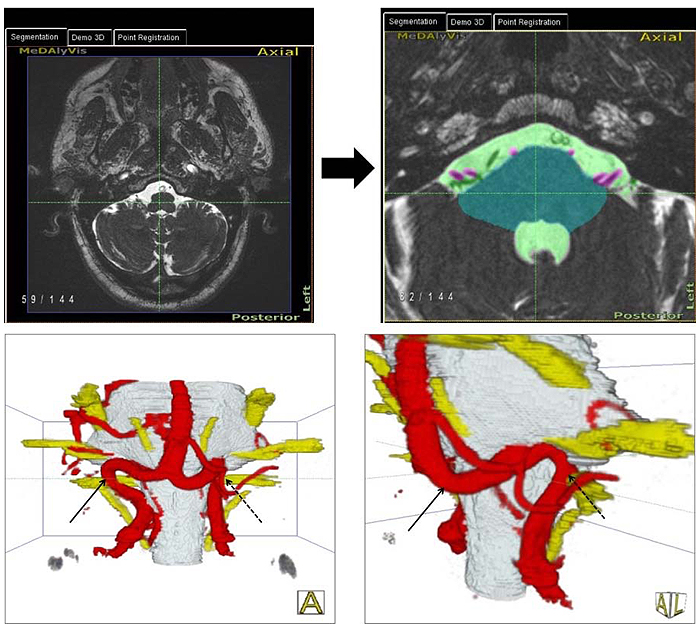
In addition, an axial T2-weighted turbo spin echo sequence (T2w TSE), coronar fluid attenuated inversion recovery (FLAIR), axial T1-weighted turbo spin echo sequence (T1w TSE) and diffusion weighted sequence (DWI) of the standard mri protocol were acquired and analyzed. The coronar FLAIR and transversal T2-weighted sequence were used to determine the degree of deep and periventricular white matter lesions (WMLs). The Fazekas score was used for the classification of WMLs [40].
2.5. Statistics
Clinical variables were compared between the two groups using a Mann-Whitney-U-Test. Over all groups, a Levene’s Test was applied to assess the equality of variances of the values. A standard level of statistical significance (p <0.05) was used and data were reported with 95% confidence intervals. The statistical analysis was performed using SPSS (IBM SPSS Statistics, Version 25).
3. RESULTS
3.1. Anatomical Results
All 44 patients underwent brain MRI and image processing was performed for all MRI images. Twenty-nine patients (66%) had evidence of NVC at the VLM. The left side was affected in 16 patients (36%) and the right side in 7 patients (16%). We observed a bilateral NVC in 6 (14%) patients. No NVC (either left or right) was seen in 15 (34%) patients (Table 1). The total number of NVC observed on the left is 22 and on the right side 13 (Table 1). According to a previously published classification [4], we found NVC type I in 16 patients, NVC type II in 4 patients and NVC type III on the left VLM in 2 patients. On the right VLM, 10 patients had an NVC type I, 2 patients a NVC type II and 1 patient a NVC type III.
Patients were separated into two groups characterized by cases with left NVC including bilateral NVC (“NVC left all”) and all cases without NVC on the left including cases showing NVC right (“No NVC left”); (Table 1). Overall, we had 22 cases as “NVC left all” and 22 cases as “no NVC left”.
Women were affected in 4 of the 22 cases of left NVC and men in 18 cases. Similar results were found in the group without NVC left with 4 women and 18 men; there was no difference between gender and the detection of left NVC (χ2 = 0.00, p = 1.0).
3.2. Comparison of Clinical Data
3.2.1. Comparison of Body Characteristics, Behavior and Lifestyle
We analyzed age, BMI, alcohol consumption, sporting activity, nicotine consumption in pack-years and the duration of hypertension. Two patients lacked information about their smoking behavior. The group variances in age were not homogeneous (p=0.018) (Table 2).
There was a significant difference in age between the two groups (median NVC left all= 57, no NVC left = 63; p=0.034; (Table 3a and Fig. 2). There were no significant differences among the two groups in gender, medical history, duration of HTN (NVC left all: 164.55 months, no NVC left: 202.68 months), smoking (NVC left all: 8.63 months, no NVC left: 15.71 months) and alcohol consumption (NVC left all: 152.27 g/week, no NVC left: 181.02 g/week) (Table 3a).
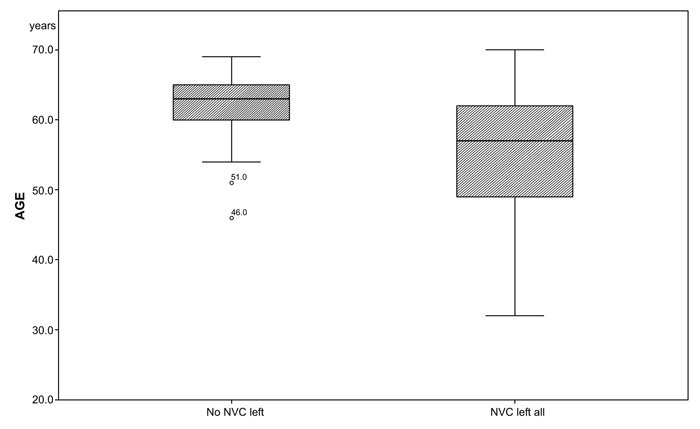
Groups | Prevalence | Percent in % |
Left NVC | 16 | 36.40 |
Right NVC | 7 | 15.90 |
Bilateral NVC | 6 | 13.60 |
No NVC | 15 | 34.10 |
NVC left all (left NVC + bilateral NVC) | 22 | 50.00 |
No NVC left (no NVC + right NVC) | 22 | 50.00 |
- | Levene’s -Test for equality of Variance | ||
F | Significance | ||
AGE | Equal variance assumed | 6.116 | 0.018 |
Equal variance not assumed | |||
Daily diastolic BP | Equal variance assumed | 4.370 | 0.043 |
Equal variance not assumed | |||
Nighttime systolic BP | Equal variance assumed | 1.028 | 0.317 |
Equal variance not assumed | |||
Nighttime diastolic BP | Equal variance assumed | 17.085 | 0.000 |
Equal variance not assumed | |||
Nighttime Mean arterial pressure | Equal variance assumed | 7.236 | 0.010 |
Equal variance not assumed | |||
Day- and nighttime systolic BP | Equal variance assumed | 2.324 | 0.135 |
Equal variance not assumed | |||
Day- and nighttime diastolic BP | Equal variance assumed | 9.704 | 0.003 |
Equal variance not assumed | |||
Day- and nighttime Mean arterial pressure | Equal variance assumed | 5.898 | 0.020 |
Pulse wave velocity | Equal variance assumed | 0.12 | 0.912 |
Equal variance not assumed |
Parameters | NVC left | N | Mean | Std.-deviation | P Value |
Patients specific body characteristics, behavior and lifestyle (3a): | - | - | - | - | - |
AGE (years) | Yes | 22 | 56.00 | 9.68 | 0.034 |
No | 22 | 61.32 | 5.63 | ||
BMI (kg/m2) | Yes | 22 | 29.20 | 3.09 | 0.944 |
No | 22 | 29.48 | 4.08 | ||
Alcohol consumption (g/week) | Yes | 22 | 152.27 | 138.85 | 0.509 |
No | 22 | 181.02 | 151.41 | ||
Sporting activity (hours/week) | Yes | 22 | 2.22 | 2.7287 | 0.162 |
No | 22 | 1.341 | 2.4562 | ||
Nicotine pack years | Yes | 20 | 8.63 | 13.39 | 0.309 |
No | 22 | 15.71 | 20.37 | ||
Duration of hypertension (months) | Yes | 22 | 164.55 | 118.19 | 0.301 |
No | 22 | 202.68 | 143.10 |
Comparison of BP and vascular parameters (3b): | NVC left | N | Mean | Std.-deviation | P Value |
Casual systolic BP (mmHg) | Yes | 22 | 158.10 | 19.65 | 0.614 |
No | 22 | 161.68 | 19.41 | ||
Casual diastolic BP (mmHg) | Yes | 22 | 94.86 | 12.81 | 0.823 |
No | 22 | 93.96 | 11.18 | ||
Casual Heart Rate (bpm) | Yes | 22 | 66.14 | 15.61 | 0.778 |
No | 22 | 64.77 | 11.83 | ||
Casual syst. BP after 3 min Standing (mmHg) | Yes | 22 | 158.10 | 17.64 | 0.725 |
No | 22 | 159.36 | 17.31 | ||
Casual diast. BP after 3 min. Standing (mmHg) | Yes | 22 | 95.18 | 11.74 | 0.953 |
No | 22 | 95.36 | 9.76 | ||
Casual Heart Rate after 3 min. Standing (mmHg) | Yes | 21 | 65.71 | 13.19 | 0.313 |
No | 22 | 69.32 | 14.58 | ||
Daytime systolic BP (mmHg) | Yes | 21 | 144.19 | 16.30 | 0.465 |
No | 22 | 147.50 | 11.64 | ||
Daytime diastolic BP (mmHg) | Yes | 21 | 88.86 | 11.69 | 0.971 |
No | 22 | 88.64 | 7.09 | ||
Daytime Mean arterial Pressure (mmHg) | Yes | 21 | 108.48 | 12.69 | 0.473 |
No | 21 | 109.86 | 7.60 | ||
Daytime Heart Rate (bpm) | Yes | 21 | 68.62 | 10.95 | 0.381 |
No | 22 | 69.27 | 11.94 | ||
Nighttime systolic BP (mmHg) | Yes | 21 | 128.71 | 18.73 | 0.155 |
No | 22 | 135.64 | 14.23 | ||
Nighttime diastolic BP (mmHg) | Yes | 21 | 76.10 | 13.76 | 0.331 |
No | 22 | 77.41 | 5.70 | ||
Nighttime Mean Arterial Pressure (mmHg) | Yes | 21 | 94.71 | 14.81 | 0.199 |
No | 21 | 97.91 | 8.01 | ||
Nighttime Heart Rate (bpm) | Yes | 21 | 61.33 | 8.73 | 0.913 |
No | 22 | 61.36 | 9.81 | ||
Day- and Nighttime syst. BP (mmHg) | Yes | 21 | 139.29 | 15.96 | 0.243 |
No | 22 | 144.14 | 10.80 | ||
Day- and nighttime diast. BP (mmHg) | Yes | 21 | 84.95 | 11.90 | 0.697 |
No | 22 | 85.32 | 5.96 | ||
Day- and nighttime Mean arterial Pressure (mmHg) | Yes | 21 | 104.14 | 12.76 | 0.284 |
No | 21 | 106.33 | 6.61 | ||
Day- and nighttime Heart Rate (bpm) | Yes | 21 | 66.43 | 9.94 | 0.488 |
No | 22 | 66.96 | 10.96 |
Comparison of ECG data (3c) | NVC left | N | Mean | Std.-Deviation | P Value |
Heart Rate (bpm) | Yes | 22 | 59.50 | 10.43 | 0.934 |
No | 22 | 59.23 | 10.43 | ||
QT | Yes | 22 | 427.32 | 31.61 | 0.613 |
No | 22 | 422.46 | 23.06 | ||
Sokolov-Lyon-Index | Yes | 22 | 2.59 | 0.8485 | 0.259 |
No | 22 | 2.26 | 0.8156 | ||
Cornell-Index | Yes | 21 | 1.96 | 0.7807 | 0.125 |
No | 22 | 1.59 | 0.5525 |
Comparison of Echocardiographic Data (3d) | NVC left | N | Mean | Std.-Deviation | P Value |
Posterior Wall Thickness Diastolic (mm) | Yes | 21 | 11.62 | 1.21 | 0.11 |
No | 21 | 10.79 | 1.67 | ||
Posterior Wall Thickness Systolic (mm) | Yes | 21 | 18.04 | 1.95 | 0.545 |
No | 21 | 17.68 | 2.40 | ||
Septum Thickness Diastolic (mm) | Yes | 21 | 12.76 | 1.55 | 0.344 |
No | 21 | 12.16 | 2.21 | ||
Septum Thickness Systolic (mm) | Yes | 21 | 18.04 | 2.26 | 0.457 |
No | 21 | 17.44 | 3.24 |
Comparison of Cardiac MRI data (3d) | NVC left | N | Mean | Std.-Deviation | P Value |
Left ventricular wall (in grams) | Yes | 22 | 148.50 | 25.20 | 0.606 |
No | 20 | 149.25 | 37.69 | ||
Right ventricular wall (in grams) | Yes | 19 | 40.79 | 8.83 | 0.754 |
No | 20 | 40.48 | 8.73 |
Pulse Wave Analysis (3e) | NVC left | N | Mean | Std.-Deviation | P Value |
Peripheral systolic Pressure (mmHg) | Yes | 22 | 156,41 | 23,38 | 0,581 |
No | 22 | 159,27 | 21,51 | ||
Peripheral Diastolic pressure (mmHg) | Yes | 22 | 91,50 | 12,83 | 0,597 |
No | 22 | 93,23 | 11,51 | ||
Peripheral Mean Pressure (mmHg) | Yes | 22 | 115,36 | 15,93 | 0,69 |
No | 22 | 117,00 | 14,77 | ||
Peripheral Augmentation Index (%) | Yes | 22 | 33,50 | 10,75 | 0,778 |
No | 22 | 35,64 | 8,03 | ||
Aortic Systolic Pressure (mmHg) | Yes | 22 | 148,36 | 23,70 | 0,511 |
No | 22 | 151,82 | 21,19 | ||
Aortic diastolic Pressure (mmHg) | Yes | 22 | 92,55 | 12,97 | 0,69 |
No | 22 | 94,14 | 11,95 | ||
Aortic augmentation pressure (mmHg9 | Yes | 22 | 20,09 | 10,45 | 0,581 |
No | 22 | 20,77 | 7,73 | ||
Aortic augmentation index (%) | Yes | 22 | 25,46 | 10,06 | 0,596 |
No | 22 | 27,55 | 7,65 | ||
Pulse wave velocity | Yes | 17 | 9,97 | 1,71 | 0,987 |
No | 18 | 10,18 | 1,91 |
3.2.2. Comparison of BP, ECG and Vascular Parameters
The systolic casual BP in patients with left NVC was measured with a mean value of 158.10 (SD 19.65) mmHg in comparison to the cases without NVC left with 161.68 (SD 158.10) mmHg. In the variances of the measurements of 24-h-BP, statistical significances could be found especially in the night measurements between the two group; nighttime diastolic BP (NVC left all: 76.10 mmHg, SD 13.76; no NVC left all: 77.41mmHg, SD 5.7; p<0.001), nighttime mean arterial pressure (MAP) (NVC left all: 94.71 mmHg, SD 14.81; no NVC left all: 97.91 mmHg, SD 8.01; p=0.010), day- and nighttime diastolic BP (NVC left all: 84.95 mmHg, SD 11.90; no NVC left: 85.32 mmHg, SD 5.96; p=0.003) and day- and nighttime MAP (NVC left all: 104.14 mmHg, SD 12.76; no NVC left: 106.33 mmHg, SD 6.61; p=0.020), (Figs. 3 and 4).
24-h-BP measurements and office systolic and diastolic BP showed no statistical differences between the two groups (Tables 3b and 3c). One patient did not participate in the 24-h-BP measurements.
3.2.3. Comparison of Echocardiographic and Cardiac MRI Data
No differences were found between the two groups in any of the cardiac MRI and echocardiographic data with a posterior wall thickness of 11.62 mm diastolic in the group of left NVC all and 10.79 mm in the group without NVC left (Table 3d). The systolic posterior wall thickness was 18.04 mm in NVC left all and 17.68 mm in no NVC left similar. We obtained cardiac MRI data in 42 patients. The right ventricular wall could be measured only in 39 patients due to artifacts.
3.2.4. Comparison of Pulse Wave Analysis (PWA) and Pulse Wave Velocity (PWV)
There were no significant differences among the two groups in the measurements of PWA and PWV (Table 3e). Pulse wave velocity was measured in 35 patients.
3.2.5. Data on Antihypertensive Medication
Table 4 presents the medication profile between the two groups, where no differences could be found. In the group “NVC left all”, 12 patients received an ACEI’s and 12 patients and ARB’s. A combination of both were given only in 2 cases with left NVC all. In the group without left NVC, 11 patients received ACEI’s, 14 patients ARB’s and a combination in 3 cases.
All patients received thiazide type diuretics. 18 cases of left NVC all received CCB and only 8 cases received CCB in the group no NVC left. Beta-blockers were given to 15 patients with left NVC and 9 patients without NVC. Central sympatholytic drugs were given to six patients with NVC left and to four patients without NVC.
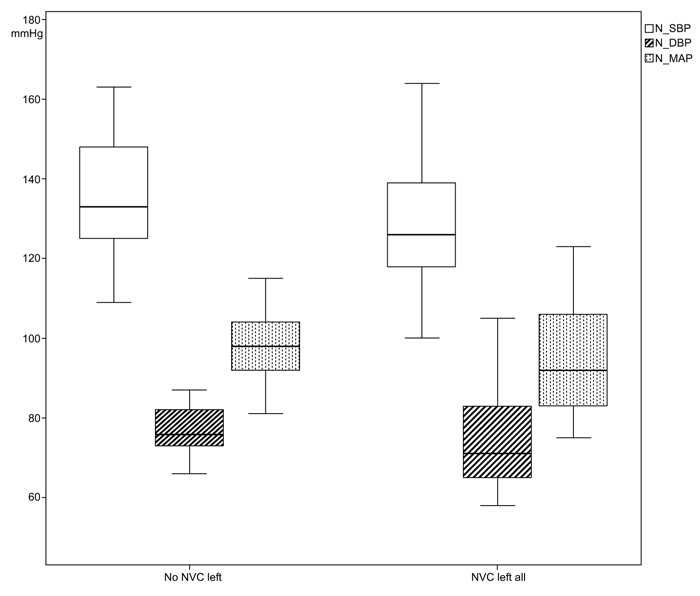
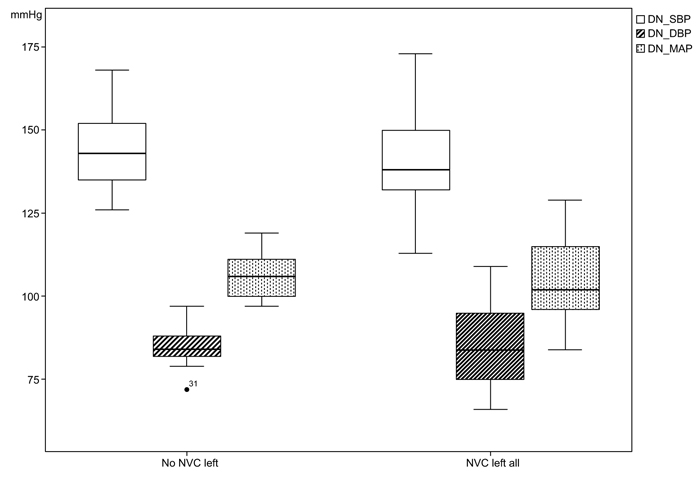
Pharmacologic Management | NVC Left All | No NVC Left |
ACEI‘s | 12 | 11 |
ARB‘s | 12 | 14 |
Combination of ACEI’s and ARB’s | 2 | 3 |
Thiazide type diuretics | 22 | 22 |
CCB | 18 | 8 |
Beta-blockers | 15 | 9 |
Central symphatholytic drugs | 6 | 4 |
Alpha-blockers | 2 | 5 |
3.2.6. Analysis of WMLs
WMLs could be found in 11 cases in NVC left, 9 of those with moderate findings (Score 1) and 2 patients with severe findings (Score 2). In the group without NVC, we found WMLs in 14 cases, of those 10 with moderate findings (Score 1) and 4 with severe changes (Score 2) according to Fazekas.
4. DISCUSSION
In this study, we tried to identify specific patterns of clinical and hemodynamic data of hypertensive patients which may indicate the presence of an NVC at the VLM. MVD can be an option for therapy in patients with severe HTN refractory to medical therapy [41]. The long term results after MVD in patients with essential HTN and NVC show that surgery could be a successful alternative therapy in certain subgroups of patients with HTN [3].
Several studies have already demonstrated an association between NVC and elevated sympathetic activity [10, 19, 42, 43]. An elevated sympathetic activity is associated with an elevation of left ventricular mass [33, 44]. Our data showed no significant difference between hypertensive patients with or without NVC in the chamber sizes and functions obtained by CMR and echocardiography. This could be explained by the nearly equal duration of HTN in both groups (p=0.301). LVH appears in all types of HTN independent of the respective cause.
Correlation between vessel contact with the left retroolivary sulcus and higher plasma norepinephrine concentrations with depressor response to clonidine support the theory that vascular contact on the VLM may contribute to neurogenic mediated essential HTN [19].
Our group cohorts were similar in regard to gender differences. The same number of women and men were affected in both groups, with a higher frequency of men.
The structural changes regarding the WMLs in brain MRI were also similar in the two groups.
The significantly lower mean age (median: NVC left all= 57; no NVC left all=63; p=0.034) and greater variance (p=0.018) in the group NVC left all indicate that NVC can appear in younger patients and may develop faster into a treatment resistant HTN. Patients in the group “no NVC left”, demonstrate a more homogenous age distribution, (Fig. 2).
From the 24-h-BP measurements, we could identify a statistically significant difference of variance for daytime (p=0.020) and nighttime diastolic BP (p<0.001) as well as mean arterial pressure (p=0.020) between both groups and not interindividual as previously reported [21], (Figs. (3 and 4). This might be explained by a still existing autonomic regulation of BP in cases with NVC.
We could not find any significant differences between the two groups for PWA and PWV. This might be explained by the nearly similar duration of HTN in both groups. Organ damage is the same in all types of HTN, independent of the cause of HTN.
Since JANNETTA’s first suggestion of an association between arterial HTN and NVC of the left VLM and the REZ of the ninth and tenth cranial nerve (N.IX and N.X), a series of imaging studies with controversial results were published [6, 7, 10-16, 41, 43, 45, 46]. The meta-analysis of BOOGAARTS et al. [47] showed, a statistical significant higher prevalence of NVC at the left VLM in essential HTN as compared to normal control subjects. At this point, one may question whether all these studies are comparable, because some of them are retrospective [13, 16] and some of them are non-blinded studies [7, 16]. Several further aspects have to be taken into consideration in the meta-analysis. The first aspect is the precise definition and localization of NVC at the VLM and which structures are involved. Some studies did not give a precise description of how they define NVC and the respective region [11, 16]. A detailed anatomical examination of the NVC at the left VLM was published in an anatomic cadaveric study and described three distinct types of NVC associated with HTN [4]. Another aspect is the different quality of imaging. Some studies used high-resolution MRI and others used standard T1- or T2-weighted images with 3-5 mm slice thickness [7, 11, 13, 16, 45]. Nowadays, high resolution MR-sequences like CISS in combination with high resolution MR-Angiography (TOF) are widely accepted and considered as the appropriate sequences for evaluating the relationship between cranial nerves and vessels in the posterior cranial fossa [48-50]. One major aspect is the difference between prospective and retrospective studies and the quality of patient data collection regarding HTN. In our study, all patients received multiple workups over several years.
With the method used in this study, we introduced an advanced technique and standard for the evaluation of neurovascular relationships especially for the detection of NVC in essential HTN. With the use of this technique, it is possible to obtain 3D-presentations of NVC in HTN, which are reproducible.
Using 2D slice images as an exclusive source of information, an extensive experience on the part of the observer is required to achieve a correct assessment of the relationship of vessels and cranial nerves in the posterior cranial fossa. Hyperactive cranial nerve dysfunction syndromes require a comprehensive 3D analysis [38]. In this study, we used for the first time an advanced technique of image processing to generate 3D visualizations of the posterior cranial fossa in patients with HTN [39]. This was achieved through explicit segmentation of the cerebrospinal fluid (CSF), the brain stem and the cranial nerves, followed by implicit segmentation and separation of the vessels and cranial nerves embedded in CSF. Lastly, 3D visualizations were generated. The highest quality of the 3D visualization was achieved mostly through fusion of the CISS- and TOF- data. With this technique, nearly all the technical artifacts that may appear in this region, like the hemodynamic pulsation in the CSF were eliminated. Using these steps of image processing as previous introduced [48] and described [38, 39], we were able to have an exact and comprehensible anatomical representation of each patient (Fig. 1).
The literature affords robust anatomical and physiological evidence that compression of REZ of CN IX-X and adjacent VLM – especially on the left side, is known to convey inputs from the baroreceptors of the (left-sided) cardiac atrium – could be the origin of systemic arterial HTN [29]. JANNETTAS’ results were confirmed in several surgical studies [1-3, 29, 51] with short and long term results.
NVC of the VLM has also been detected in brachydactyly associated with HTN [52]. The latest genetic studies on HTN and its association with brachydactyly strongly suggest that mutations in PDE3A are responsible for HTN by contributing to a general increase in peripheral vascular resistance in this syndrome [53]. Until now, we have no data regarding PDE3A from our patients.
Previous reports showed that compression of VLM was closely associated with BP variability in the subacute ischemic stroke phase [21]. Elevated BP is present in approximately 80% of patients with ischemic strokes [54]. As with intracranial aneurysms, a wide range of BP variability seems to be also relevant for NVC in HTN [55, 56]. Further studies are necessary to find possible correlations.
The small number of patients could be considered as a limitation of our study. However, this could be explained by highly selective criteria for diagnosis of treatment resistant HTN as well as the availability of all clinical and hemodynamic parameters. Further large and detailed clinical studies will be needed to elucidate the clinical factors to influence the interaction between increased BP variance and the detection of NVC at the left VLM.
CONCLUSION
NVC of the VLM can be detected in patients with arterial HTN. Young patients with arterial, treatment resistant HTN should be examined for the presence of NVC at the VLM before permanent organ damage occurs. The possible association of NVC in HTN and the detailed analysis of 24 h BP regarding the BP variability should be further evaluated. Clinical and hemodynamic parameters do not sufficiently indicate the presence of NVC. Possible candidates for MVD can only be identified after confirming a positive finding of NVC with the use of MRI.
AUTHORS' CONTRIBUTION
All authors contributed to the study's conception and design. Material preparation, data collection and analysis were performed by Panagiota MANAVA, Ramin NARAGHI and Peter HASTREITER. The first draft of the manuscript was written by Panagiota MANAVA and all authors commented on the previous versions of the manuscript. All authors read and approved the final manuscript.
ETHICS APPROVAL AND CONSENT TO PARTICIPATE
This study was approved by the ethics committee of the University Erlangen-Nuremberg (FAU) in Erlangen, Germany with the ethical approval number: 2633.
HUMAN AND ANIMAL RIGHTS
No animals were used in this research. All human research procedures followed were in accordance with the ethical standards of the committee responsible for human experimentation (institutional and national), Declaration of Helsinki and the guidelines for “good clinical practice” (GCP).
CONSENT FOR PUBLICATION
Each patient provided written informed consent prior to inclusion in this observational study.
AVAILABILITY OF DATA AND MATERIALS
The data supporting the findings of the article is available from corresponding author [P.M] upon reasonable request.
FUNDING
This research did not receive any specific grant from funding agencies in the public, commercial, or not-for-profit sectors.
CONFLICT OF INTEREST
We wish to confirm that there are no known conflicts of interest associated with this publication and there has been no financial support for this work that could have influenced its outcome.
ACKNOWLEDGEMENTS
This article is dedicated to Peter J. Jannetta (*April 05. 1932 - †April 11. 2016) for his pioneer work on neurovascular compression at the ventrolateral medulla and arterial hypertension.