All published articles of this journal are available on ScienceDirect.
The Neural Processing of Second Language Comprehension Modulated by the Degree of Proficiency: A Listening Connected Speech fMRI Study
Abstract
While the neural network encompassing the processing of the mother tongue (L1) is well defined and has revealed the existence of a bilateral ventral pathway and a left dorsal pathway in which 3 loops have been defined, the question of the processing of a second language (L2) is still a matter of debate. Among variables accounting for the discrepancies in results, the degree of L2 proficiency appears to be one of the main factors. The present study aimed at assessing both pathways in L2, making it possible to determine the degree of mastery of the different speech components (prosody, phonology, semantics and syntax) that are intrinsically embedded within connected speech and that vary according to the degree of proficiency using high degrees of prosodic information. Two groups of high and moderate proficiency in L2 performed an fMRI comprehension task in L1 and L2. The modifications in brain activity observed within the dorsal and the ventral pathways according to L2 proficiency suggest that different processes of L2 are supported by differences in the integrated activity within distributed networks that included the left STSp, the left Spt and the left pars triangularis.
INTRODUCTION
Over 50% of the world’s population has been reported to be bilingual (i.e., theoretically presenting equal fluency in two languages). As a consequence, many studies have investigated the way multiple languages are processed in the brain [1]. While the neural network encompassing the processing of the mother tongue (L1) has been fairly consistent, the question of the processing of a second language (L2) has long been a matter of debate. In fact, numerous functional neuroimaging studies contend that differential networks process L1 and L2 [2-10] whereas other studies have failed to reveal differences in the cerebral networks underlying L1 and L2 [11]. These discrepancies have been mainly accounted for by several environmental factors, such as age of acquisition, which predicts that the later a second language is acquired, the more the neural recruitments of L2 and L1 will differ. Though supported by a few neuroimaging studies [7, 12, 13], this hypothesis has been contradicted by other investigations that failed to show differences in brain activity for L1 and L2 [14-17]. However, it could be argued that the degree of attained proficiency is a much more important factor than the age of acquisition per se [6] and that this degree of proficiency accounts for the potential differences in the neural network(s) involved in the processing of L1 and L2. This issue has been addressed by a small number of neuroimaging studies focusing on a specific language speech component and that have shown that a lesser proficiency in late L2 learners seems to be associated with less overlapping neural substrates compared to the relatively fixed network involved in L1 [5, 16-20].
Indeed, the claim to high proficiency in a second language actually entails the ability to master simultaneously the linguistic, pragmatic and affective structures of speech (using the phonological, syntactic, semantic and prosodic components which are intrinsically overlapping). The connected speech stimuli technique presents the advantage of working in all three directions and appears to be the most ecological method since it requires the listener to master the 4 main speech components. Moreover, many studies have claimed that speech perception precedes speech production [21, 22]. The notion of phonological deafness [23, 24] supports this view as it states that a sound must be adequately perceived so that it can be adequately produced. Among the few existing investigations using short-story listening tasks and proficiency, an fMRI study addressing the question of the neural network underlying L1 and L2 in L2 moderately-proficient subjects revealed that a limited extent of activation in L2 may reflect heterogeneous and inconsistent patterns of neural activation [19]. Two PET studies have specifically addressed the question of the neural network underlying L1 and L2 among low and highly L2-proficient subjects [5, 17]. These studies revealed that highly L2-proficient subjects exhibited the same pattern of activation for L1 and L2, which encompassed the bilateral Superior Temporal Gyrus (STG), whereas the less proficient subjects presented reduced activations in the bilateral STG for L2.
However, the model of a bilateral dorsal-ventral auditory speech processing in L1, with a preferential left-sided involvement has emerged from many convergent studies [25-28]. This model posits: (1) a ventral pathway [29-31] and a dorsal pathway [32]. More particularly, within the dorsal stream, three different loops (involved in phonology, semantics and complex sentences) related to speech have been revealed [33]. Each of these loops has a starting point in the left parietal or temporal areas and finally projects to frontal areas due to its connection via the arcuate fasciculus [34].
Assessing both pathways during L2 auditory speech comprehension is of considerable interest since it allows for determining the degree of mastery of L2 according to L2 proficiency in a wide language network. The aim of the present study is therefore to address the question of differences between the neural networks, including the ventral and dorsal pathways, elicited by presentation of expressive connected speech stimuli in French (L1) and English (L2). Using functional Magnetic Resonance Imaging (fMRI) and connected speech stimuli with high degrees of prosody, two groups of late learners of L2 with moderate and high proficiency are compared.
MATERIALS AND METHODS
Subjects
Twenty-eight right-handed French late learners of English (aged 22-43 years, mean 31 years ± 7.02) participated in the study after having given their informed written consent in accordance with the guidelines approved by the Ethics Committee of the Bordeaux Medical University. So as to constitute a group of high proficiency in English, half of the subjects were recruited among English teachers and researchers having just returned from Anglophone countries. The subjects constituting the group of moderate proficiency in English were recruited among students in biology and the university staff. Both groups were homogeneous with respect to handedness (all right-handed), sex (7 males, 7 females), age of L2 acquisition (mean age 11 years old, ± 0.5), means of L2 acquisition (formal instruction in primary and secondary school) and level of education (from Bachelor’s degree to Bachelor’s degree + 5, no significant difference between the two groups, p <0.887).
Subjects had no hearing or neurological disorders and normal structural MRI scans. They had no prior experience of either behavioral or fMRI tasks and were not familiar with the stimulus materials.
Pre-Scanning Aural Dictation Test: High and Moderate English Proficiency Groups
Subjects’ proficiency was assessed by a discrimination task commonly used in the Foreign Language Department of Bordeaux University. This task, an aural English dictation, which aims at assessing the global percentage of French students’ general perception of English, allowed us to confirm the a priori determination of both groups. Results were analyzed by two senior lecturers in English blind to the experiment as follows: the number of words correctly perceived by each subject was counted and divided by the number of words in the extract (spelling errors were not taken into account). A t-test revealed a significant difference between the two groups (p< 0.001) with highly proficient L2 subjects presenting a global perception of the English extract ranging from 90 to 99% (mean 94,6 %, ± 3.02), whereas moderately proficient L2 subjects presented a global perception ranging from 50 to 70 % (mean 59,67 %, ±5.46).
Stimuli
Two English and French 90-s-long prosodic connected speech stimuli were recorded by trained native speakers in a soundproof room at a 16 bits/44.1 kHz sampling rate. The English stimulus recording was an extract of “The open boat” by Stephen Crane [35] , and the French one was an extract which was similar to the English one in terms of level of language, style and prosody (“Et si c’était vrai”, by Marc Lévy), [36]. Both the English and French 90-s-long recordings were digitally cut at sentence boundaries to obtain 3 fragments of 30-s-long activation periods for each language. So as to avoid any disturbing noise, the 5 ms of the beginning of each fragment were gradually increased (fade-in process) and the 5 ms of the end of each fragment were gradually decreased (fade-out process).
fMRI Procedure
The fMRI protocol consisted in 6 thirty-second-long stimuli placed within 7 fifteen-second-long rest periods, the total length of the procedure being 4 minutes and 45 seconds. Participants were asked to listen to the stimuli while remaining motionless and to keep their eyes closed. The speech stimuli were presented binaurally through headphones specifically designed for use in the scanner (MR Confon, Magdeburg, Germany). The English and French stimuli were not crossed purposely so as to prevent subjects from switching from one language to the other. However, the language stimuli order was counterbalanced across subjects (within each group).
Post-Scanning Aural Dictation Test
After the scanning session, subjects were exposed to an aural dictation, called ‘post-scanning test’, corresponding to the L2 stimuli presented in the scanner. This task allowed for determining the global percentage of French students’ general perception of English but also allowed for classifying the percentage of mistakes according to syntactic, phonetics and lexical errors. For example in the sentence “There was a considerable silence as the boat bumped over the furrowed sea to deeper water”, if the word “bumped” was not written, it was scored as a lexical error, if the word “furrowed” was written “furrow” it was scored as a phonetic error and if the word “as” was written “has” it was scored as a syntactic error. Each error was only counted once. Results were analyzed by two senior lecturers in English blind to the experiment. Statistical analyses were performed by t-tests.
fMRI Acquisition
The MRI data were collected at 1.5 Tesla using an Intera Philips system (Philips Medical System, Best, Netherlands) equipped with an eight-element phased-array head coil. For each subject, a series of 95 functional scans were acquired using a T2*-weighted single shot echo-planar sequence (FOV = 256x256, Matrix = 128x128, TR/TE = 3000/58 ms, Flip angle = 90°, SENSE factor = 2). Each scan included 25 slices (no gap, thickness 4mm) parallel to AC-PC (Anterior Commissure-Posterior Commissure). Three dummy scans were used to reach steady-state magnetization. A high-resolution T1-weighted anatomic scan was also acquired to obtain a morphological reference (25 slices parallel to AC-PC with a resolution of 1x1x4 mm3, no gap).
Whole Brain Analyses in L2 and L1
All data were analyzed using SPM2 (Statistical Parameter Mapping, Wellcome Department of Imaging Neuro-science, London UK) and MATLAB 7.1 (The Mathworks Inc., Natick, MA, USA) and SPSS 16.0 (SPSS, Chicago).
For each individual subject, the dynamic scans were adjusted for slice timing differences, realigned to the first scan to correct for head movement, normalized to the standard Montreal Neurological Institute space (MNI) and spatially filtered by applying an 8 mm3 Gaussian kernel. High-pass filtering (cut off 128s) was performed to remove low frequency artifacts. Then, a general linear model was used to model the data [37]. The functional time series were modeled by a boxcar model convoluted with a canonical hemodynamic response. After estimation of the model parameters, two linear contrasts, English-Rest and French-Rest, were applied to the parameter estimates to test the effects of the English (L2) and French (L1) listening conditions.
These two contrasts per participant were then entered in a second-level within-subjects ANOVA analysis to allow inference at the population level for each group (high proficiency, moderate proficiency). First, a conjunction analysis was performed to determine the activated areas in both L1 and L2 [38]. Then, the differences between L1 and L2 were identified by contrasting English-Rest with French-Rest. Clusters of activated voxels were identified with a global height threshold of p < 0.05 FDR-corrected for multiple comparisons and an extent threshold of 100 voxels. Anatomical localization was performed using the AAL atlas [39].
ROIs Analyses in L2
To compare the behavior of the brain areas potentially involved in the ventral and dorsal pathways for L2 processing across groups (highly and moderately-proficient subjects), an ROI analysis was conducted. Each ROI was defined as an 8 mm-diameter sphere centered in published coordinates from a meta-analysis [33] and from a general paper on dorsal and ventral streams [27], (Table 1). Then, a Pearson correlation test was performed to assess the strength between BOLD signal and scores of the after scanning test (global perception percentage, syntactic, phonetics and lexical mistakes percentage).
Coordinates of Centre Voxels of the Bilateral Ventral Stream and the Left Dorsal Stream, the Left 3 Loops and the T1 a Common to the 3 Loops
Location | MNI Coordinates | x | y | z |
---|---|---|---|---|
Left Ventral stream | STSp | -50 | -54 | 22 |
MTG (T2ml) | -57 | -37 | 1 | |
ITG (pole) | -47 | 6 | -24 | |
Right Ventral stream | STSp | 50 | -54 | 22 |
MTG (T2ml) | 57 | -37 | 1 | |
ITG (pole) | 47 | 6 | -24 | |
Left Dorsal stream | Spt | -54 | -38 | 12 |
F3opd | -49 | 16 | 24 | |
F3tv | -44 | 26 | 2 | |
Loop for phonology | SMG | -42 | -52 | 37 |
F3td | -44 | 23 | 15 | |
Loop for semantics | AG | -45 | -68 | 26 |
preF3opd | -42 | 4 | 36 | |
Loop for complex sentences | STSp | -50 | -54 | 22 |
F2p | -37 | 10 | 48 | |
Common area | T1a | -57 | -13 | -8 |
Note: STSp refers to posterior Superior Temporal Sulcus, MTG to Middle Temporal Gyrus, ITG to Inferior Temporal Gyrus, Spt to Sylvian parieto-temporal, T1a to anterior superior temporal sulcus, SMG to Superior Marginal Gyrus, AG to Angular Gyrus, preF3opd to dorsal preF3 opercularis, F3tv to ventral F3 triangularis, F3td to dorsal F3 triangularis, F3opd to dorsal F3 opercularis, F2p to posterior F2.
Ventral Pathway
Three ROIs that have been associated in the ventral pathway per hemisphere i.e., the posterior Superior Temporal Sulcus (STSp), the Middle Temporal Gyrus (MTG or T2ml) and the Inferior Temporal Gyrus (ITG or pole) were symmetrically selected. Data were extracted for each participant and entered in an ANOVA analysis with Hemisphere (2) and ROI (3) as within-subject factors and Group (2) as a between-subject factor. Post-hoc analyses were performed by t-tests.
Dorsal Pathway
Three ROIs that have been associated in the dorsal path-way per se for the left hemisphere, i.e. 1 temporo-parietal ROI (Spt), 2 frontal ROIs: 1 pars opercularis (F3opd), 1 pars triangularis (the ventral part of the pars triangularis, i.e., F3tv) were selected.
To further investigate the dorsal pathway by determining the degree of mastery of different speech components within L2-connected speech processing across the 2 groups, 7 supplementary left ROIs were selected based on the assumption advanced by Vigneau and collaborators [33] concerning the existence of 3 distinct loops, each of them connected by the arcuate fasciculus, involved in either phonology, semantics or complex verbal materials. For the phonological loop, 1 parietal ROI (SMG) and 1 frontal pars triangularis ROI (the dorsal part of the pars triangularis, i.e., F3td) were selected, for the semantics loop 1 parietal ROI Angular Gyrus (AG) and 1 frontal pars opercularis ROI (preF3opd) were selected and for the complex sentences loop 1 frontal ROI in the dorsal part of the middle frontal gyrus (F2p) was chosen (the STSp having already been selected for the ventral pathway). Since the anterior superior temporal gyrus (T1a) has been revealed to be activated in all three loops, this specific region was also selected. Data were extracted for each participant and entered in an ANOVA analysis with ROI (10) as within-subject factor and Group (2) as a between-subject factor. Post-hoc analyses were performed by t-tests.
Functional Connectivity Analysis in L2
A supplemental functional connectivity analysis aiming at identifying brain areas functionally correlated with the starting point of the left dorsal pathway (left Stp) was performed for each group. First, data were controlled for extensive movements. No subject was excluded from the subsequent analysis since movements were lower than 1mm and 1° in any of the x, y, z directions. Then, the brain voxels time series were normalized to the same mean intensity and restricted to the 2m30s-long-period corresponding to the English listening stimulus. Next, a temporal correlation analysis was performed for each subject with a simple regression model in which each brain voxel time course was regressed against the left Stp reference time course. This reference time course was built with the voxels time courses extracted from the left Stp ROI defined above which were projected onto the first eigenvector of that ROI to create a summary time course. The resulting parameter map for this correlation for each subject was then entered in a group-level analysis to demonstrate brain areas which were functionally connected with the left Stp (one sample t-test, p <0.01 FDR-corrected with an extent threshold of 100 voxels).
RESULTS
Post-Scanning L2 Aural Dictation Test
A t-test revealed a significant difference between the two groups for L2 (p< 0.001) with highly-proficient L2 subjects presenting a global perception of the English extract ranging from 92 to 99% (mean 96.7 %, ± 1.93), whereas moderately-proficient L2 subjects presented a global perception ranging from 50 to 67 % (mean 60.1 %, ±4.56). For syntax, a t-test revealed a significant difference between the two groups for L2 (p< 0.001) with highly-proficient L2 subjects presenting a success rate ranging from 96 to 100% (mean 98.8%, ± 1.09), whereas moderately-proficient L2 subjects presented a success rate ranging from 74 to 86 % (mean 78.3 %, ±3.6). For phonetics, a t-test revealed a significant difference between the two groups for L2 (p< 0.001) with highly-proficient L2 subjects presenting a success rate ranging from 98 to 100% (mean 98.8%, ± 0.77), whereas moderately-proficient L2 subjects presented a success rate ranging from 80 to 91 % (mean 87.85 %, ±3.18). For lexicon, a t-test revealed a significant difference between the two groups for L2 (p< 0.001) with highly-proficient L2 subjects presenting a success rate ranging from 98 to 100% (mean 99%, ± 0.55), whereas moderately-proficient L2 subjects presented a success rate ranging from 90 to 97 % (mean 93.9 %, ±2.05).
Whole-Brain Analyses in L2 and L1
Conjunction Analyses
Whatever the groups (high or moderate), common activated areas between second and native languages were observed bilaterally in the superior temporal gyrus (STG, BA 22) and the middle temporal gyrus (MTG, BA 21), and in the left cerebellum. Moreover, for highly proficient L2 subjects, supplementary areas such as the bilateral ITG (BA 38), the bilateral IFG (BA 44) and the right cerebellum were activated by both second and native languages (Fig. 1, Table 2).
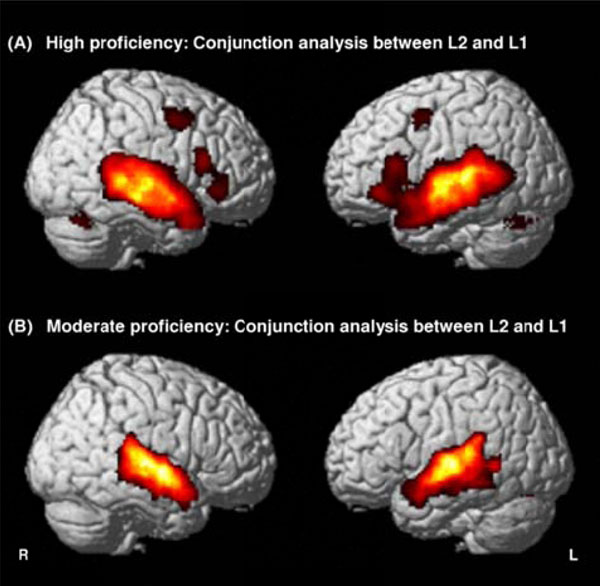
Conjunction maps between English (L2) and French (L1) contrasts revealing common areas sharing the same activity between the two languages in highly proficient L2 subjects (A) and in moderately proficient L2 subjects (B). Threshold at P<0.05, FDR-corrected for multiple comparisons with a spatial extent of at least 100 voxels.
Conjunction Maps between L2 and L1 in L2 Highly and Moderately Proficient Subjects
Brain area | K | T max | Location (MNI coordinates) | ||
---|---|---|---|---|---|
x | y | z | |||
L2 and L1 in highly proficient subjects | |||||
Left MFG | 149 | 5.50 | -44 | 2 | 48 |
Left STG | 7659 | 35.53 | -58 | -16 | -4 |
This cluster also includes subpeaks | |||||
ITG | 33.18 | -54 | -8 | -18 | |
IFG | 9.54 | -46 | 34 | -6 | |
Left cerebellum | 563 | 9.83 | -30 | -64 | -30 |
Right IFG | 513 | 7.76 | 50 | 20 | 14 |
Right MFG | 245 | 6.10 | 52 | 0 | 48 |
Right STG | 6706 | 27.40 | 62 | -4 | -12 |
This cluster also includes a subpeak | |||||
ITG | 25.70 | 62 | -40 | -12 | |
Right cerebellum | 713 | 12.02 | 28 | -68 | -24 |
L2 and L1 in moderately proficient subjects | |||||
Left STG | 4266 | 19.39 | -58 | -10 | -4 |
Left cerebellum | 466 | 7.23 | -22 | -62 | -32 |
Right STG | 4140 | 24.82 | 60 | -6 | -4 |
Note: MFG refers to Middle Frontal Gyrus, IFG to Inferior Frontal Gyrus, STG to Superior Temporal Gyrus, ITG to Inferior Frontal Gyrus. Threshold at P <0.05, FDR-corrected for multiple comparisons with a spatial extent of at least 100 voxels, K referring to the cluster size in voxels. The T maxima and MNI coordinates are for the peak activated voxel in each cluster.
L1 High vs. L1 Moderate Analyses
Whatever the contrast (L1 High > L1 Moderate, or L1 Moderate > L1 High), no brain areas were found to be significantly more activated for the native language.
L2 > L1 Analyses
Contrast analysis for both the highly- and moderately-proficient L2 groups did not reveal any significant brain areas which would be more activated for the second language as compared to the native language (Table 3).
L1 vs. L2 in L2 Highly and Moderately Proficient Subjects
Brain area | K | T max | Location (MNI coordinates) | ||
---|---|---|---|---|---|
x | y | Z | |||
L1-L2 moderately proficient subjects | |||||
Left ITG | 222 | 7.62 | -58 | -4 | -20 |
Right ITG | 243 | 8.45 | 52 | -14 | -20 |
L2-L1 moderately proficient subjects | |||||
ϕ | |||||
L1-L2 highly proficient subjects | |||||
Ø | |||||
L2-L1 highly proficient subjects | |||||
ϕ |
Note: Threshold at P <0.05, FDR-corrected for multiple comparisons with a spatial extent of at least 100 voxels, K referring to the cluster size in voxels. The T maxima and MNI coordinates are for the peak activated voxel in each cluster.
L1>L2 Analyses
Whereas no brain area was more activated in the native language as compared to the second language for the highly-proficient L2 group, the moderately-proficient L2 group revealed significantly greater activation in the ITG (BA 38) and the middle temporal lobe (BA 22-21) bilaterally for their native language as compared to their second language (Table 3).
ROIs Analyses in L2
Ventral Pathway
Concerning the ventral pathway, there was a statistically significant main effect of Group (F(1,26) = 54.66, p<0.001, and a statistically main effect of ROI (F(2,25) = 50.11, p<0.001) whereas no significant main effect of Hemisphere was revealed. However, these results were qualified by a significant interaction of Group x ROI (F(2,25) = 5.36, p<0.006), a significant interaction of ROI x Hemisphere (F(2,25) = 3.34, p<0.05), and a significant interaction of Hemisphere x ROI x Group (F(5,25) = 4.62, p<0.02). No significant differences were revealed for Hemisphere x Group. Follow-up t-tests revealed that the 3 left ROIs as well as their right counterparts were significantly more activated in the highly proficient L2 group as compared to the moderately proficient L2 group (left STSp, p<0.001; left MTG, p<0.001 and left ITG p<0.001, right STSp, p<0.001, right MTG, p<0.001 and right ITG, p<0.001).
Dorsal Pathway
Concerning the dorsal pathway and the 3 loops, there was a main effect of ROI (F(9,18) = 14.04, p<0.001) and a main effect of Group (F(1,26) = 48.917, p<0.001. These results were qualified by a significant interaction of ROI x Group (F(9,18) = 7.55, p<0.006). Follow-up t-tests (Fig. 2) revealed that the 3 ROIs pertaining to the dorsal pathway per se were significantly more activated in highly-proficient subjects than in moderately-proficient subjects (Spt, p<0.001; F3opd, p <0.002 and F3tv p<0.001). Concerning the loops, 4 ROIs were significantly more activated in the highly-proficient group as compared to the moderately-proficient group: the 2 ROIs pertaining to the loop for the processing of complex sentences (STSp, p <0.001, F2p, p<0.002), the frontal ROI pertaining to the phonological loop (F3td, p<0.001) and the temporal ROI common to the three loops, T1a, (p <0.001). The 3 other ROIs, i.e., the 2 ROIs pertaining to the semantic loop (AG and preF3opd) and the temporal ROI (SMG), revealed no significant difference between the 2 groups.
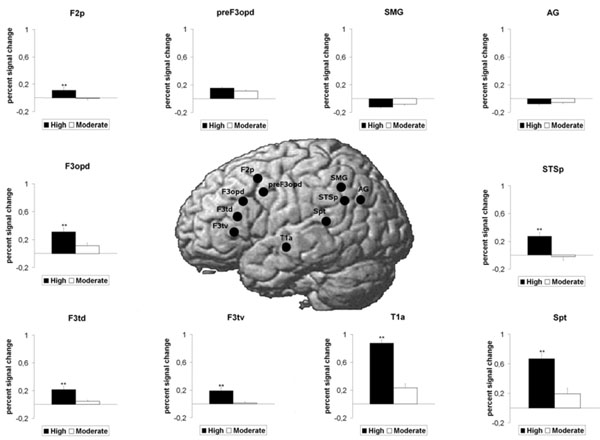
BOLD response increase for the highly proficient L2 subjects as compared to the moderately proficient L2 subjects in ROIs pertaining to the dorsal pathway as well as the three loops for the English stimulus (L2). Mean standard errors bars are indicated for each ROI. Double asterisks (**) above histogram bars indicate a statistically significant difference between the 2 groups (P<0.01).
Correlations between ROIs Analyses and Post Scanning Aural Dictation Test
Ventral Pathway
Statistical analyses using Pearson correlation revealed that BOLD activation in the left and right STSp, T2ml and pole presented a significant positive correlation coefficient not only with the score of the global perception but also with the score of syntax, phonetics and lexicon (Table 4).
Bivariate Correlations between Success Scores of the after Scanning Aural Dictation and both Pathways
Ventral pathway | ||||
---|---|---|---|---|
% Global perception | Syntax | Phonetics | Lexicon | |
ROIs | sig | |||
LSTSp | 0,609* * | 0,600* * | 0,544* * | 0,597* * |
RSTSp | 0,715* * | 0,662* * | 0,683* * | 0,775* * |
LT2ml | 0,584* * | 0,620* * | 0,516 * * | 0,432* |
RT2ml | 0,573* * | 0,572* * | 0,526* * | 0,495* * |
Lpole | 0,631* * | 0,620* * | 0,574* * | 0,621* * |
Rpole | 0,612* * | 0,612* * | 0,584* * | 0,469* |
Dorsal pathway | ||||
% Global perception | Syntax | Phonetics | Lexicon | |
ROIs | sig | |||
LSpt | 0,611* * | 0,602* * | 0,546* * | 0,597* * |
LF3opd | 0,540* * | 0,545* * | 0,510* * | 0,428* |
LF3tv | 0,682* * | 0,677* * | 0,621* * | 0,624* * |
Phonological loop | ||||
LSMG | 0,356 | 0,385 | 0,359 | 0,163 |
LF3td | 0,804* * | 0,754* * | 0,817* * | 0,733* * |
Semantics loop | ||||
LAG | 0,120 | 0,119 | 0,147 | 0,010 |
preF3opd | 0,053 | 0,051 | 0,086 | 0,041 |
Complex sentences loop | ||||
STSp | 0,609* * | 0,600* * | 0,544* * | 0,597* * |
F2p | 0,422* | 0,411* | 0,372 | 0,446* |
T1a | 0,660* * | 0,672* * | 0,659* | 0,436* |
sig: Pearson’s correlation coefficient
* * significant at 0.01 level
* significant at 0.05 level
Dorsal Pathway
Statistical analyses using Pearson correlations revealed that BOLD activation in the 3 ROIs pertaining to the dorsal pathway per se (i.e., the left Spt, F3opd and F3tv) were positively correlated not only with the score of the global perception but also with the score of syntax, phonetics and lexicon.
Concerning the loops, BOLD activation in the 2 ROIs pertaining to the complex sentence loop, i.e., STSp and F2p presented a significant positive correlation with the score of syntax. BOLD activation in the F3td ROI pertaining to the phonological loop presented a significant positive correlation with the score of phonetics, whereas BOLD activation in the other ROI, SMG, was not significantly correlated with behavioural data. BOLD activation in the 2 ROIs pertaining to the semantic loop were not significantly correlated with behavioural data, whereas BOLD activation in the temporal ROI common to the three loops, T1a, presented a significant positive correlation with the score of syntax, phonetics and lexicon (Table 4).
Functional Connectivity Analysis in L2
The functional connectivity analysis in highly-proficient L2 subjects revealed that not only the bilateral temporal lobes (STSp, MTG, ITG) but also the left articulatory loop (BA 6- 44) and its right counterpart were significantly correlated with the starting point of the left dorsal pathway (left Spt) during L2-connected speech listening. Conversely, moderately proficient L2 subjects exhibited only a significant correlation between the left Spt and the bilateral STG and MTG as well as the bilateral BA 6 (Fig. 3, Table 5).
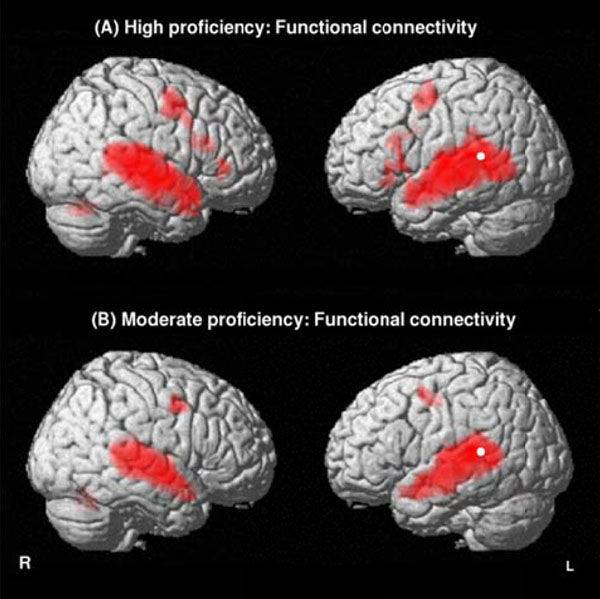
Functional connectivity identifying brain areas functionally correlated with the starting point of the left dorsal pathway (left Spt) for the English stimulus (L2) in highly proficient L2 subjects (A) and in moderately proficient L2 subjects (B). Threshold at P<0.01 FDR-corrected for multiple comparisons with a spatial extent of at least 100 voxels.
Functional Connectivity in L2 Moderately and Highly Proficient Subjects
Brain area | K | T max | Location (MNI coordinates) | ||
---|---|---|---|---|---|
x | y | Z | |||
L2 highly proficient subjects | |||||
Left MFG | 532 | 14.79 | -44 | -4 | 50 |
This cluster also includes a subpeak | |||||
IFG | 8.14 | -42 | 4 | 30 | |
Left STG | 8126 | 38.59 | -56 | -14 | -6 |
This cluster also includes subpeaks | |||||
ITG | 15.22 | -46 | -2 | -18 | |
IFG | 9.54 | -46 | 34 | 26 | |
Left cerebellum | 321 | 5.76 | -22 | -56 | -24 |
Right MFG | 277 | 7.60 | 58 | -2 | 50 |
Right IFG | 171 | 7.10 | 40 | 22 | 24 |
Right STS | 4841 | 17.97 | 68 | -14 | -6 |
Right MTG | 114 | 6.07 | 40 | -50 | -4 |
Right cerebellum | 625 | 9.83 | 32 | -66 | -32 |
L2 moderately proficient subjects | |||||
Left STS | 4910 | 28.05 | -58 | -12 | -8 |
Left cerebellum | 133 | 7.49 | -26 | -60 | -32 |
Right STS | 3776 | 21.54 | 62 | -8 | -8 |
Right cerebellum | 307 | 8.21 | 28 | -62 | -32 |
Note: Threshold at P <0.01, FDR-corrected for multiple comparisons with a spatial extent of at least 100 voxels, K referring to the cluster size in voxels. The T maxima and MNI coordinates are for the peak activated voxel in each cluster.
DISCUSSION
This investigation examined brain mechanisms underlying the processing of connected prosodic speech comprehension in moderately- and highly-proficient late second language learners. A main finding was that L1 and L2-connected prosodic speech stimuli were found to share the same neural network encompassing both the dorsal and ventral pathways in highly-proficient L2 subjects. This finding is in accordance with previous studies [14-17]. On the contrary, moderately-proficient L2 subjects presented no significant activation in the whole ventral pathway while processing L2 and L1, since they only exhibited common activations in the STS and the MTG. These results also concord with the PET study by Perani [5] which revealed that while L1 elicited bilateral activations in the STS and left activation in the IFG, not only did L2 clearly induce less activation in the bilateral STS (which was circumscribed to the posterior and middle STS bilaterally) but it also induced less activation in the left IFG due to the poor L2 mastery. In a related study, Dehaene [19] used an auditory fMRI paradigm at the sentence level in moderately-proficient subjects in L2 and observed less activation and higher inter-subject variability for L2 than for L1 in the left STG. As it has been argued that the temporal poles are crucial to sentence comprehension [33, 40] and are multimodal association areas, it is likely that syntactic, semantic and episodic information is integrated in this brain area to transform the language input into meaningful representations. In the case of language comprehension, the temporal poles could implement propositionalization, i.e. the process required for combining words into semantically based content units [41]. This process clearly uses prosodic, syntactic and lexical information to derive a semantic representation [42]. Moreover, temporal activity has been shown to increase with the linguistic complexity of the stimulus [43, 44]. The lower activation observed in this region in L2 relative to L1 in moderately-proficient L2 subjects could indicate that these subjects failed to combine the linguistic, pragmatic and affective structures of L2 speech stimuli.
A second important finding concerns the fact that L2 entails more activation in both the ventral and dorsal path-ways and in the dorsal right counterpart in highly-proficient subjects as compared to moderately-proficient subjects. Concerning the ventral pathway, the 3 ROIs of STSp, MTG and ITG were found to be significantly more activated bilaterally in the L2 highly-proficient group than in the moderately-proficient L2 group. Furthermore, significant positive correlations between these 3 ROIs and the score of global perception as well as the score of syntax, phonetics and lexicon revealed that the more these brain structures are activated, the more accurately subjects performed the tasks. Taken together, it can be hypothesized that moderately-proficient subjects have difficulties in meeting the required task, suggesting that the degree of language proficiency seems to be a critical factor in shaping the neuronal organization of language [15, 17]. The reduced activation of the MTG could reflect the absence of highly automatized and efficient processes of acoustic phonological processing in L2 as compared to L1, since the MTG has been postulated to be involved in sentence evaluation and sentential integration [45]. As for the STSp, it has been reported to be involved in the processing of the semantic integration of complex linguistic material in L1 [46, 47] and more particularly in the linkage of linguistic structures to meaning [48, 49]. The lesser involvement of the STSp in the L2 moderately-proficient group could mean that these subjects are less successful in extracting specific cues from the L2 stimuli to relate them to meaning.
Concerning the dorsal pathway, which is strongly left-dominant, this sensory motor loop provides the functional anatomic basis for verbal working memory [50], i.e., the ability to use articulatory-based processes (rehearsal) to keep auditory based representations (storage) active. It appears that neither the Spt area nor its projections in BA 44 (F3opd, F3tv) were significantly recruited by the moderately proficient L2 subjects, which would mean that these subjects failed to recruit the dorsal stream in the same way as highly proficient L2 subjects. The Spt area can be considered as a transitional zone between the perception and semantic integration of L1 auditory speech stimuli and is involved in either phonology or semantics [33]. Moderately proficient L2 subjects’ abilities to decipher phonological cues and transform them into semantic units were less developed than in the highly-proficient L2 subjects. The ultimate projections to Broca’s area were also significantly less recruited in the moderately-proficient subjects as compared to the highly-proficient subjects, a finding likely due to their difficulties in recruiting the working memory system. This assumption is supported by the functional connectivity analysis which revealed that the left pars triangularis (F3td, F3tv) and its right counterpart were significantly correlated with the starting point of the left dorsal pathway (left Spt) in highly-proficient subjects, whereas no correlation between the left pars triangularis (Broca’s area) and the left Spt was observed in moderately-proficient subjects. The latter result is in accordance with previous work suggesting a functional connection between the auditory cortex and the prefrontal associative cortex involved both in the retrieval and rehearsal of auditory information and in auditory working memory in L1 [44, 51-56]. Moreover, the significant correlation between the right BA 44 and the left Stp in highly proficient subjects (and the lack of correlation in moderately-proficient subjects) is of considerable interest since this right area was also revealed to be more activated in our highly-proficient L2 subjects. This area has been reported to be involved in durational processes and in temporal analyses [57-60]. More specifically, rhythm analysis tasks have revealed either a bilateral prefrontal activation (BA 6-44), [59] or a right lateralized activation [55, 61, 62]. In addition, this specific region has been reported to be involved in the recognition of the so-called "emotional prosody" [63-66]. Taken together, this could mean that highly-proficient L2 subjects present a mastery of the prosodic dimension which would be the basis underlying the linguistic dimension, whereas moderately-proficient L2 subjects would miss this prosodic basis.
Concerning loops within the dorsal pathway, it has been postulated that a loop for semantics would involve the Angular Gyrus (AG) and a part of the inferior frontal gyrus (pre pars opercularis, PrF3op). The AG could be the site where semantic associations are made, whereas PrF3op could be the region where the selection among semantic knowledge elicited by task requirements is computed [67]. Interestingly, our two groups did not demonstrate any differences in activation in both the AG and the PrF3op, suggesting that semantics is equally acquired for each group, which would mean that one of the first speech components acquired in L2 is semantics. This is in accordance with an fMRI study reporting that lexical-semantic processing seems to be the easiest speech component mastered in L2 acquisition [9]. A loop for phonology would involve the Supra Marginal Gyrus (SMG) and a part of the inferior frontal gyrus (pars triangularis, F3td) [33]. Interestingly, no differences were found between our two groups for the SMG, but the F3td frontal region revealed a highly significant reduction of activation in moderately-proficient L2 subjects. The left SMG is associated with phonological processing [68, 69] and has recently been viewed as the starting point of the working memory loop for phonology which then projects frontally [33]. This would mean that moderately-proficient L2 subjects could analyze the acoustic cues as being speech sounds, but were unable to combine these phonemes into phonological units, which can be inferred from the lower activity in F3td. This assumption is supported by the significant positive correlation between F3td and the global perception score as well as with the phonetics score. A third loop dedicated to the processing of complex sentences may involve the posterior Superior Temporal Sulcus (STSp) and the posterior middle frontal gyrus (F2p), and it is believed to be involved in the integration of complex verbal material underlying the comprehension of complex sentences, dialogues and connected speech. Not surprisingly, both areas (STSp and F2p) revealed a highly significant reduction of activation in moderately-proficient L2 subjects as compared to highly-proficient L2 subjects. In fact, moderately-proficient L2 subjects would miss altogether the grammatical and syntactic information of the L2-connected speech stimulus and only process the semantic information, a possibility which is supported by the significant positive correlation between these 2 brain regions, STSp and F2p, and the global perception and syntactic scores.
GENERAL CONCLUSION
This study demonstrates that in the case of high L2 proficiency, L2 and L1 share the same neural network encompassing the ventral pathways bilaterally and the dorsal pathway, whereas moderately-proficient L2 subjects fail to recruit the dorsal pathway and some brain areas involved in the ventral pathway bilaterally in L2 relative to L1. Moreover, concerning the processing of L2 auditory comprehension, moderately-proficient L2 subjects presented less activation in the dorsal pathway and in the loops devoted to complex sentence comprehension and phonology, as well as in some brain areas encompassing the ventral pathway as compared to highly-proficient L2 subjects. The modifications in brain activity within the dorsal and the ventral pathways according to second language proficiency strongly suggest that different processes of the second language are supported by differences in the integrated activity within distributed networks that include the left STSp, the left Spt and the left pars triangularis. Since the present investigation restricted the analysis of L2 processing to two groups of moderate and high degrees of proficiency, an interesting future study could manipulate the degree of proficiency by assessing a continuum from L2 beginners to L2 bilinguals, which would allow for a better characterization of the gradual mastering of L2.
FUNDING
The research was supported by INSERM (contrat d'interface INSERM/Université Victor Segalen to IH) and Pôle Aquitaine Santé to MA.
CONFLICT OF INTEREST
None declared.
ACKNOWLEDGEMENTS
The authors would like to thank Dr. Anne-Marie Carassou and Dr. Ray Cooke for editing the English version of the manuscript.