All published articles of this journal are available on ScienceDirect.
Hippocampal Morphology in a Rat Model of Depression: The Effects of Physical Activity
Abstract
Accumulating in vivo and ex vivo evidences show that humans suffering from depression have decreased hippocampal volume and altered spine density. Moreover, physical activity has an antidepressant effect in humans and in animal models, but to what extent physical activity can affect hippocampal volume and spine numbers in a model for depression is not known.
In this study we analyzed whether physical activity affects hippocampal volume and spine density by analyzing a rodent genetic model of depression, Flinders Sensitive Line Rats (FSL), with Magnetic Resonance Imaging (MRI) and ex vivo Golgi staining.
We found that physical activity in the form of voluntary wheel running during 5 weeks increased hippocampal volume. Moreover, runners also had larger numbers of thin spines in the dentate gyrus. Our findings support that voluntary wheel running, which is antidepressive in FSL rats, is associated with increased hippocampal volume and spine numbers.
INTRODUCTION
Imaging studies have demonstrated structural brain changes in depression in hippocampus, amygdala, striatum, frontal cortex and ventricles. Consistently, a volume loss of the hippocampal formation is detected using MRI [1-3] and it appears that it is the time with depression that determines the extent of shrinkage of hippocampus [4]. Moreover, depression is associated with cognitive deficits and postmortem analyses show hippocampal atrophy [5, 6]. The total number of neurons is not altered but it is the size of the neuropil and glial cell numbers that are reduced [7].
Chronic stress or sustained high levels of glucocorticoids leads to reduction and retraction of dendritic spines of hippocampal pyramidal neurons in animal models [8]. Similarly, post mortem analysis of depressed patients also show reduction of hippocampal volume and retraction of dendritic spines of hippocampal pyramidal neurons [7].
A non-pharmacological intervention suggested to treat depression is physical training. Indeed, it is now widely accepted that physical training has antidepressant properties in both humans [8-12] and in animal models including the FSL rats [13-18]. The FSL rat is a selectively bread rat model of depression that displays several depressed behaviors [19]. It is even noted that many of the effects observed in FSL rats after chronic treatment with selective serotonin reuptake inhibitors (SSRIs), such as decreased immobility time in the forced swim test, increased hippocampal neurogenesis and levels of the neuropeptide Y (NPY), are also observed after long-term wheel running in FSL rats [20-22]. In other models of voluntary exercise, in rodent models, it has also been noted that the cellular as well as the dendritic structure of the hippocampus can be altered [23-30].
However, even though rats respond similarly to for example SSRI and wheel running, the underlying mechanism for antidepressant pharmacotherapy and physical activity is under investigation [31].
Interestingly, physical activity increases hippocampal volume in both humans and rodents [32-34]. However, to the best of our knowledge it is only in the preadolescent (developing) human brain that the hippocampus increased by exercise [33]. Whether exercise can alter hippocampal volume in a rat model of depression in post adolescent individuals is not known.
Others have studied dendritic spines in the hippocampus, and the relation between stress and exercise. It has been noted, in different rodent models, that voluntary exercise increases the number of spines in the hippocampus [28, 29, 35, 36].
In this study, we tested whether voluntary wheel running could alter hippocampal volume and synaptic spines in FSL rats.
The animals were group housed and bred at Karolinska Institutet in standard cages until the start of the experiment. All animal experiments were approved by the Ethical committee for animal research in Stockholm, Sweden. The average age of the animals at the start of the experiment was three months. The animals had free access to food and water and were housed in a controlled environment of 12-h light/dark cycle.
During the whole experiment, male FSL rats were single housed in cages (43 cm x 22 cm x 20 cm) with and without free access to a running wheel (34 cm in diameter) (n = 30 for both groups) during a period of five weeks. After the five week period the animals were used for either an in vivo MRI experiment or for an ex vivo golgi staining experiment. Running distance was recorded and stored every tenth minute by a computer-based data system with customized software, which registers revolutions. Similarly as in previous studies the rats gradually increased daily running distance during the first week and then leveled out the daily running during the rest of the experiment [14, 16, 22, 37]. On average over the whole 5 week period the runners covered a daily distance of 1250 ± 146 m (n=30) per day (Fig. 1). Previously we have shown that wheel running is neurogenic and antidepressive [14, 16, 22, 37]. Now we tested if voluntary wheel running also can induce volumetric changes of hippocampus and ventricles as well as spine numbers in dentate gyrus (DG) in the depressed rat strain.
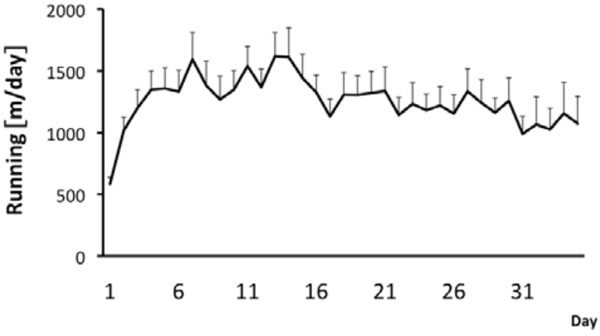
Running behaviour in FSL rats. The runners had continuous access to the running wheels during 5 weeks. Values are mean running distance/day±SEM (n=30).
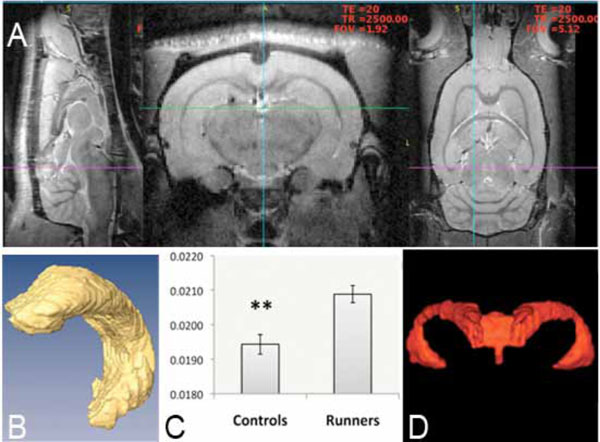
MRI-based 3-D analysis of hippocampus at 9.4 T. Images (A) show a brain from a sagital, axial and coronal view. Volumetric analysis of segmented hippocampus (B) and whole brain gave an increase in hippocampal/total brain size when comparing runners to nonrunners (control) (C). No change was noted in ventricular volume and a representative image (D), which is showing most frontal parts of the ventricular system is displayed. Values are mean hippocampus/total brain ±SEM (**, P < 0.01, unpaired two-tailed Student’s t-test, n=15).
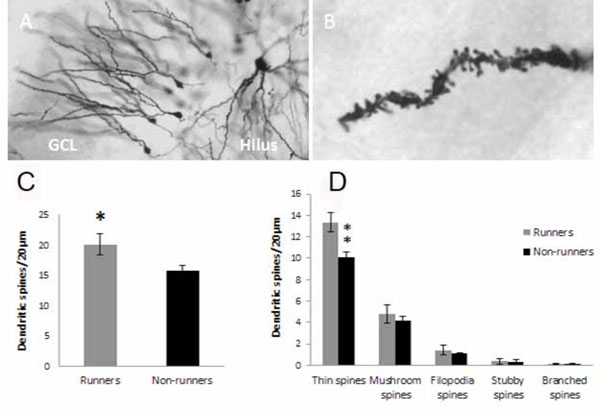
Spine analysis on dentate gyrus neurons. Golgi impregnated granule neurons in the granule cell layer (GCL) of hippocampus at low (A) and high magnification (B) were traced. Spine density and type of spine were analyzed on a distance of 20µm from the end of the dendrite. Thin, mushroom, filopodia, stubby and branched spines could be distinguished (Ziess microscope, 100 x objective with a numerical aperture on 1.4)(B). Running for five weeks increased spine density in the dentate gyrus of hippocampus (C). The increased density was found in the population of thin spines (D). ** p<0.01 and *p<0.05 comparing runners vs non-runners Unpaired two-tailed Student´s t-test.
Animals (n = 7 runners and n = 8 controls) were subjected to MRI scanning within 2 days after blocking access to the running wheels. The in vivo MRI experiments were conducted using a horizontal 9.4 T Varian magnet equipped with a 12 cm inner diameter gradient system with maximum gradient strength of 600 mT/m. The gradient coils have capacity for 8 shim channels (Z, X, Y, Z2, XZ, YZ, XY and X2Y2). An actively tuned, circularly polarized birdcage resonator with an inner diameter of 72 mm and a resonator length of 100 mm was used for excitation (Rapid Biomedical, Wurzburg, Germany). A linearly polarized ‘rat brain’ 4-channel phased array surface coil with mean coil diameter 39 mm, resonator length 26.5 mm (Rapid Biomedical, Wurzburg, Germany) served as receiving coil. Animals were anaesthetized with isoflurane (5% for induction and maintained at 1.75% during scanning) in a 1:9 mixture of O2 and air. Rats were positioned in a prone position in a MR compatible animal holder with the head firmly fixed. The receiving surface coil was secured to the animal holder above the head of the rat.
The volumetric 3D-data were acquired using an inversion recovery 3d fast spin echo sequence, with TR 1500 ms, TI 500 ms, ETL 8, kzero 1, matrix size 512x128x128 field of view (FOV) 51.2x22x19.2 mm3. Three saturation bands were employed to suppress signal from tissue outside of the FOV. Total acquisition time was 1h 25min. Temperature was maintained at 37±0.2 ºC throughout the MRI experiment with a feedback controlled air-heater system. In addition, respiration rate, pulse and oxygen saturation was monitored (SA Instruments) during scanning. At the end of the imaging experiment the animals were sacrificed with an i.p. overdose of sodium pentobarbital.
The left hippocampus and the whole brain for each rat was manually segmented on the 3D dataset using AMIRA software (Amira 3.1, Template Graphics Software, Inc., San Diego, California) by a blinded experimenter. The volume was quantified based on the size of the voxel and the numbers of voxels comprising the hippocampus or the brain. To adjust for possible differences in body and brain size in runners versus non-runners, the ratio hippocampus: whole brain was calculated. Data was subsequently analyzed with Student’s t-test. 3-D image segmentation of the ventricles volumes was performed with ITK-SNAP version 2.2 (http://www.itksnap.org), an open source segmentation software [38].
Volumetric measurements on 3D MRI images of left hippocampus and whole brain revealed that voluntary running during five weeks significantly increased the hippocampus/whole brain ratio (0.0194±0.0003, non-runners (n=8) versus 0.0209±0.0002, runners (n=7); t= 3.61, d.f.=13, **p<0.01, 2-tailed unpaired Student´s t-test) (Fig. 2A-C) but had no effect on the ventrical/brain ratio (0.00671±0.000217, non-runners (n=8) versus 0.00650±0.000150, runners (n=7); t=0.79, d.f.=13, p= 0.44, 2-tailed unpaired Student’s t-test) or on the total brain volume (2109.6±12.1 mm3, non-runners (n=8) versus 2113.7±50.1 mm3, runners (n=7); t=0.11, d.f.=13, p=0.913 2-tailed Student’s t-test) (Fig. 2D). Thus the increased size of hippocampus (Fig. 2A-C) appears to be independent of changes in total brain and ventrical volume (Fig. 2C-D).
Given the increased hippocampal volume we next investigated whether running also could impact smaller structures of the hippocampus such as the dendritic spines. Dendritic spine numbers was analyzed in hippocampal granule cells in the dentate gyrus after five weeks of wheel running (runners, n = 7) and compared to the non-runners (n = 8).
Animals were sacrificed and the brains were rapidly removed, and placed in 5ml of Golgi-solution (A+B solution, FD Rapid Golgi kit, MTR Scientific) for 19 days. The brains were then put in solution C (FD Rapid Golgi kit, MTR Scientific) for 24h where after the brains were cut in 200µm sections using a vibratome. These sections were stained according to the manufacturers protocol (FD Rapid Golgi kit, MTR Scientific) and the neurons were traced using the NeuroLucida program (MBF Bioscience). The neurons traced (6 neurons/animal) were all at the same level (coordinates: -2.76mm from Bregma) and all around the same location in the dentate gyrus. Number and type of spine were counted and analyzed on 20µm from the very end of a dendrite (in average 3.7 sections à 20µm/neuron), as indicated in Fig. (3), using a Zeiss microscope and a 100 x objective with a numerical aperture (NA) = 1.4. Spines were defined as headless (thin) or headed protrusions (mushroom). Long thin headless protrusions (> twice the length of a thin spine) were defined as filopodia spines. Protrusions connected in the same base were defined as branched spines. Stubby spines were defined as low (<1/2 thin spine) and wide protrusion. The analysis was performed by using the NeuroLucida explorer software (MBF Bioscience). Student’s t-test was applied to treat differences in number of spines between the two groups: runners vs non-runners.
Running induced an increase in spine numbers, which was visualized and outlined in Golgi impregnated sections (Fig. 3A-C), in dentate gyrus granule cell dendrites (20.1 ± 1.73 spines/20µm; n=6 for runners versus 15.8±0.92 spines/20µm; non-runners (n=6); t= 2.20, d.f.=10, *p<0.05 2-tailed Student´s t-test) (Fig. 3). Five different types of spines were detected (thin, mushroom, filopodia, stubby and branched spines), however, only thin spines increased in number (Fig. 3D) (13.4±0.90 spines/20µm in runners (n=6) versus 10.1±0.50 spines/20µm in non-runners (n=6); t=3.18, d.f.=10, **p<0.01 2-tailed unpaired Student´s t-test).
Our data supports a previous study where the dendritic spines of dentate gyrus in Sprague-Dawley rats (weight 200-250g.) are induced by voluntary exercise. Non-runners had approximately 7.7 spines/10µm where as runners had approximately 9.9 spines/10µm, which is similar to the FSL rats in this study, where non-runners had 7.9 ± 0.46 spines/10µm versus runners that had 10.05 ± 0.87 spines/10µm [35].
As with most other treatments for depression, the understanding of the underlying biology for the positive effects of physical activity, are still under debate. Here we show, by using MRI and volumetric analysis that wheel running in single housed FSL rats increases volume as well as spine numbers of hippocampus. Our findings are also in line with a recent report that used post mortem analysis on histological sections to show that electro convulsive shock treatment (ECT), which has shown to be antidepressive in FSL rats, increases hippocampal volume [39].
Based on our findings we hypothesize that the beneficial effects of physical activity on mood could involve structural reorganization of hippocampal circuits.
Moreover, tissue-level of glutamate is decreased in adult C57BL/6J mice that exercised [34]. The astrocytic involvement in glutamate metabolism and signaling has been shown to be altered in the FSL rat [40]. Interestingly, there is a strong link between glutamate, learning, memory, synaptic plasticity (LTP) and spine dynamics in the hippocampus and cerebral cortex. The generation and turnover of new spines is an ongoing process and it is the small new spines that are the preferential site for the LTP-induction [41]. Interestingly, Gómez-Galán [40] recently showed that there is a dysfunctional astrocytic glutamate transmission in the hippocampus of the FSL rats.
It has also been suggested that exercise is associated with cell proliferation, changes of vasculature, and cellular structure that could influence the volume of the hippocampus [25]. This might be a contributing mechanism to the expressed antidepressant effects in the FSL rats.
CONCLUSION
To summarize, depressed patients have alterations in brain structures, which are progressive with the development of the disease. Here we show that wheel running, which is antidepressive and neurogenic, also increases hippocampal volume and spine numbers. Our data is in line with the hypothesis of exercise induced synaptic remodeling in hippocampus.
CONFLICT OF INTEREST
Authors Adam Sierakowiak, Anna Mattsson, Marta Gómez-Galan, Teresa Femenía Canto, Lisette Graae, Sahar Nikko Aski, Peter Damberg, Mia Lindskog, and Stefan Brené declares no conflict of interest. Elin Åberg has employment in AstraZeneca, a for-profit company engaged in the discovery, development, manufacture and marketing of proprietary therapeutics. E. Å. does not consider that this creates any conflict of interest with the subject-matter of this paper.
ACKNOWLEDGMENTS
This work was supported by the Swedish Research Council grant no. 11642, Karolinska core facility support to Karolinska Experimental Research and Imaging Center, National Institute on Drug Abuse, National Institutes of Health-Karolinska Institutet Graduate Partnerships Program.