All published articles of this journal are available on ScienceDirect.
Exploring the Neurocircuitry Underpinning Predictability of Threat in Soldiers with PTSD Compared to Deployment Exposed Controls
Abstract
Background:
Prior work examining emotional dysregulation observed in posttraumatic stress disorder (PTSD) has primarily been limited to fear-learning processes specific to anticipation, habituation, and extinction of threat. In contrast, the response to threat itself has not been systematically evaluated.
Objective:
To explore potential disruption in fear conditioning neurocircuitry in service members with PTSD, specifically in response to predictable versus unpredictable threats.
Method:
In the current study, active-duty U.S. Army soldiers with (PTSD group; n = 38) and without PTSD (deployment-exposed controls; DEC; n = 40), participated in a fear-conditioning study in which threat predictability was manipulated by presenting an aversive unconditioned stimulus (UCS) that was either preceded by a conditioned stimulus (i.e., predictable) or UCS alone (i.e., unpredictable). Threat expectation, skin conductance response (SCR), and functional magnetic resonance imaging (fMRI) signal to predictable and unpredictable threats (i.e., UCS) were assessed.
Results:
Both groups showed greater threat expectancy and diminished threat-elicited SCRs to predictable compared to unpredictable threat. Significant group differences were observed within the amygdala, hippocampus, insula, and superior and middle temporal gyri. Contrary to our predictions, the PTSD group showed a diminished threat-related response within each of these brain regions during predictable compared to unpredictable threat, whereas the DEC group showed increased activation.
Conclusion:
Although, the PTSD group showed greater threat-related diminution, hypersensitivity to unpredictable threat cannot be ruled out. Furthermore, pre-trauma, trait-like factors may have contributed to group differences in activation of the neurocircuitry underpinning fear conditioning.
INTRODUCTION
Pavlovian fear conditioning, a type of associative learning, prepares an organism to effectively avoid, escape, or mitigate the outcomes of an imminent threat [1, 2]. In individuals with posttraumatic stress disorder (PTSD), the processes underlying fear learning appear to contribute to hyper-vigilance and persistent avoidance of perceived triggers, which are associated with traumatic events, and are hallmarks of PTSD [3]. A significant percentage of military service members return from deployment with PTSD [4-6]; for systematic review, see [7]. As such, many returning service members and veterans struggle to regulate their emotional response to environmental threats as well as internally generated thoughts, images, and sensations. There is a paucity of evidence clarifying how individuals with PTSD utilize cues to regulate emotional responses to threat. Exploring the differences in neural activation in response to unpredictable and predictable threats in military service members with PTSD may provide clarification of the underlying pathophysiology associated with both combat-related PTSD and PTSD in general.
Prior neuroimaging research suggests that fear conditioning in healthy individuals relies upon the concerted function of multiple brain regions [8, 9]. Research has identified the amygdala as being central to the neurocircuitry of fear conditioning [10], with connections to multiple regions for mediating fear expression [8]. The hippocampus plays a key role in the acquisition of contextual information associated with threat during fear conditioning [9, 11-13]. The prefrontal cortex (PFC; including the dorsolateral, dorsomedial, and ventromedial regions) supports top-down regulation of conditioned fear, which is necessary for extinction learning and regulating emotional responses to learned threat [14-19].
In a typical Pavlovian fear conditioning paradigm, an originally neutral stimulus (e.g., light or tone) is repeatedly paired with an unconditioned stimulus (UCS), such as an electric shock or loud noise that triggers an automatic emotional response (i.e., the unconditioned response; UCR). After repeated pairings of the previously neutral stimulus (conditioned stimulus; CS) and the UCS, the CS comes to elicit a conditioned response (CR) similar to the UCR. Changes in behavior during the CS serves as an index of emotional learning, while the response to the UCS can be used to evaluate emotion regulation. For example, differential responses to cues that warn (CS+) of threat vs. cues that predict safety (CS-) provide an index of anticipatory fear learning. Likewise, repeated pairings of the CS and UCS lead to a phenomena known as conditioned UCR diminution as measured by both neural activation and skin conductance response [16, 20-22]. Conditioned UCR diminution occurs when a diminished emotional response is elicited by the UCS when it is preceded by the CS (i.e., the UCS is predictable) compared to presentations of the UCS alone (i.e., the UCS is unpredictable) [16-18, 23, 24]. Thus, this diminished emotional response provides an index of one’s ability to regulate the emotional response to an aversive threat.
Evidence suggests that individuals with PTSD have intact fear acquisition and extinction learning, but fear renewal and extinction recall are often impaired [9, 25, 26]; both of which rely on regulatory interactions between the PFC, hippocampus, and amygdala [11, 27]. Other evidence suggests that civilian PTSD patients have difficulty in distinguishing between threat and safe contexts, and show greater hippocampal activation than healthy controls [28]. In veterans with PTSD, the amygdala has been implicated in anticipation of aversive stimuli including electrical shock [29] and negative images [30]. Abnormal insula activation has also been observed in both veterans and civilians with PTSD [31, 32]. In a recent study with active-duty service members preparing for deployment [33], those with PTSD symptoms showed difficulty in discriminating between predictable threat and safe cues as measured via self-report ratings and startle response, suggesting an exaggerated fear response.
Although these studies present important findings concerning the anticipatory response to the warning cues that predict threat, there is a paucity of evidence highlighting PTSD abnormities in the neurocircuitry associated with the response to the threat itself. Linnman and colleagues [32] provide evidence that compared to controls, civilians with PTSD have greater activation of the putamen and insula in response to predictable threat (i.e., electrical shock) versus omitted threat (i.e., no shock). While this finding is insightful, the study does not address how individuals respond to threat when it is predictable versus unpredictable. Prior studies using healthy individuals provide some additional insight into the neural regions expected to be involved in the threat-elicited response. For example, there is an increase in amygdala activation in response to threatening stimuli (e.g., loud white noise [18]). In addition, conditioned diminution of the amygdala to predictable threat appears to rely on functional connections with regions of the PFC [16, 18, 34]. Further, individual differences in anxiety level among healthy adults impact the threat-elicited response within the neural circuit that mediates fear-related processes [18, 24, 34].
Much less is known about the function of the underlying neurocircuitry in response to threat in individuals with PTSD. PTSD is associated with compromised functioning of the amygdala, hippocampus, and PFC [9, 19, 35]. This dysregulation implicating these regions pertains to anticipation of threat, and fear renewal and extinction recall. Only limited evidence implicates the insula in PTSD as indicated by increased activation in response to predictable threat, potentially suggesting hypersensitivity to threats when they are predictable [32]. Behavioral evidence provides some support by showing that active-duty service members with PTSD have compromised emotion-based learning, which appears be attributed to hypersensitivity to threat saliency [36]. Psychophysiology findings also suggest that PTSD is associated with hypersensitivity to threat, which may be moderated by predictability as measured by startle response to aversive auditory stimuli [37]. Therefore, at a minimum, the amygdala, insula, hippocampus, and PFC are likely to be implicated in hypersensitivity to threat in PTSD.
Given that the task used in the current study consists of auditory stimuli, activation of temporal regions is expected in that the superior temporal region contains the primary auditory cortex with projections to the medial temporal region [38]. As such, the middle temporal gyrus, with projects to the hippocampus and amygdala, is also of interest in that it is implicated in auditory expectation [39], which in individuals with PTSD, might be hyperactive in response to an aversive auditory stimulus. In addition, recent evidence suggests the inferior parietal lobe is associated with successful emotion regulation [40], and is activated in response to an aversive stimulus (shock) [41]. Based on this evidence, these regions are of particular interest to the current study.
To explore potential disruption in the fear conditioning neurocircuitry in service members with PTSD, the current study compared differences in the functional magnetic resonance imaging (fMRI) signal response to threat in active-duty U.S. Army soldiers with PTSD and healthy deployment-exposed controls (DEC). A fear conditioning task was used to investigate group differences in threat expectation (participant ratings), psychophysiology (skin conductance response), and neural activation (fMRI) to predictable versus unpredictable threat. Our primary question, “Do soldiers with PTSD use learned cues to regulate their response to a threat?” We hypothesized that the DEC group would show diminished threat-related responses to predictable versus unpredictable threat. In contrast, the PTSD group would show accentuated responses to predictable versus unpredictable threat.
MATERIAL AND METHODS
Recruitment
The present study’s protocol was reviewed and approved by the U.S. Army Medical Research and Materiel Command Institutional Review Board (USAMRMC IRB). Seventy-eight active-duty U.S. Army soldiers, with prior deployment(s) to Iraq or Afghanistan as part of Operation Iraqi Freedom and/or Operation Enduring Freedom, were recruited from Fort Rucker, AL and Fort Benning, GA to participate in the current study. In addition to word of mouth, recruitment flyers and posters were placed at behavioral health clinics and other instillation facilities frequented by soldiers. Interested soldiers contacted investigators via phone or email provided on the flyers/posters. Candidates were screened for MRI contraindication, PTSD, and traumatic brain injury history. Eligible volunteers provided written informed consent. A study physician reviewed soldiers’ electronic medical records for exclusionary medical conditions (e.g., MRI contraindications such as evidence of shrapnel). If cleared by the study physician, the soldier was contacted and scheduled for testing at the Auburn University's MRI Research Center, Auburn, AL. Participants were re-consented and further screened upon arrival at the testing site.
Participants
The PTSD group consisted of 38 participants who screened positive for PTSD (structured interview using the Clinician-Administered PTSD Scale for DSM-5, CAPS-5), negative for concussion within the last eight years (Brief Traumatic Brain Injury Screening, BTBIS; and medical records), no history of moderate-to-severe TBI, and no diagnoses of additional psychiatric conditions including psychotic, bipolar, or personality disorders (medical records). A group of 40 healthy deployment-exposed controls (DEC) were enrolled in the study with no history of moderate-to-severe TBI, screened negative for PTSD, and no psychiatric history (as evidenced by medical records and self-reporting). In addition, although the DEC group reported some PTSD symptoms (see Table 1), they did not meet the criteria for PTSD using the PTSD Checklist-5 (≥ 33) nor did they have the diagnosis in their medical records. Finally, during the screening process participants were asked to report prescription medications currently in use.
DEC (n=38) | PTSD (n=33) | ||
---|---|---|---|
DESCRIPTIVES | p | ||
Male, f (%) | 29 (76%) | 33 (100%) | .002* |
Age, M (SD) | 30.7 (6.8) | 33.6 (6.8) | .070 |
Education, M (SD) | 15.5 (2.1) | 14.0 (1.8) | .002* |
Race, f (%) | .240 | ||
Caucasian | 29 (76%) | 20 (61%) | |
African Am | 2 (5%) | 7 (21%) | |
Hispanic/Latino | 3 (8%) | 2 (6%) | |
Asian | 2 (5%) | 0 | |
Native Am | 0 | 1 (3%) | |
Other | 2 (5%) | 3 (9%) | |
Combat Exposure | 7.1 (9.5) | 28.3 (8.2) | <.001* |
Childhood Experiences | 55.3 (13.3) | 53.9 (8.9) | .595 |
PSYCHOLOGICAL HEALTH | |||
Posttraumatic Stress | 9.4 (12.8) | 47.1 (17.8) | <.001* |
Alcohol Use | 5.8 (4.9) | 9.0 (8.5) | .071 |
Depression | 32.5 (8.2) | 48.4 (14.8) | <.001* |
Anxiety | 30.6 (6.8) | 46.7 (15.2) | <.001* |
Daytime Sleepiness | 8.8 (3.3) | 12.3 (5.3) | .003* |
MEDICATION, f (%) | ∆% | ||
Antidepressants | 4 (10.5) | 9 (27.3) | 16.8 |
2 or more | 0 | 7 (21.2) | 21.2 |
Antipsychotics | 1 (2.6) | 3 (9.1) | 6.5 |
Benzodiazepines | 0 | 5 (15.2) | 15.2 |
Mood stabilizers | 0 | 0 | 0 |
Stimulant | 1 (2.6) | 1 (3.0) | 0.4 |
Hypnotic (Nonbenzodiazepine) | 0 | 5 (15.2) | 15.2 |
Anxiolytic | 0 | 1 (3.0) | 3.0 |
Opioid analgesic | 1 (2.6) | 3 (9.1) | 6.5 |
Female contraceptive | 3 (7.9) | 0 | -7.9 |
Testosterone | 1 (2.6) | 1 (3.0) | 0.4 |
PDE5 inhibitors | 0 | 1 (3.0) | 3.0 |
% Medicated | 21% | 49% | 28% |
Note. Posttraumatic Stress = PCL-5; Combat Exposure = CES; Childhood Environment = CE; Alcohol Use = AUDIT; Depression = ZDS; Anxiety = ZAS; Sleepiness = ESS. ∆% = delta (PTSD minus DEC) or group difference in percentage of medication users.
PTSD Screening and Psychological Health
The following measures were administered to participants prior to the imaging procedures: Clinician-Administered PTSD Scale (CAPS-5 [42]); PTSD Checklist-5 (PCL-5 [43]; Combat Exposure Scale (CES [44]); Childhood Family Experiences (CFE [45]); Zung Depression Scale (ZDS [46]); Zung Anxiety Scale (ZAS [47]); Alcohol Use Dependency Identification Test (AUDIT [48]); Epworth Sleepiness Scale (ESS [49]); Brief Traumatic Brain Injury Screen (BTBIS [50]). See Appendix A for more details of the measures.
Fear Conditioning Task
Two tones (700 and 1300 Hz; 10 s duration) served as the conditioned stimuli (CS) and a loud (100 dB) white-noise served as the UCS (0.5 s duration) and were presented through magnetic resonance (MR) compatible pneumatic earbuds. UCS onset occurred 0.5 s before termination of one tone (CS+) and the second tone was presented alone (CS−). Conditioned UCR diminution was assessed by comparing presentations of the UCS (i.e., threat) that were paired with the CS+ (i.e., CS+UCS, predictable threat) to presentations of the UCS alone (i.e., unpredictable threat). There were a total of 20 CS+, 20 CS−, and 20 UCS alone trials presented across the two 900 s blocks of conditioning (10 CS+, 10 CS−, and 10 UCS alone per block). The inter-trial-interval (ITI) varied from 20 to 50 s (average ITI = 30 s). The stimuli were counterbalanced and presented in a pseudorandom order such that no more than three trials of the same stimulus were consecutively presented.
UCS Expectancy
UCS expectancy was used as a measure of conscious expectation of the UCS as described by prior work [51]. The present analysis focused on learning-related changes in the response to predictable vs. unpredictable threat (i.e., conditioned UCR diminution). Therefore, contrasts were limited to predictable (i.e., CS+UCS) and unpredictable (i.e., UCS alone) presentations of the UCS. E-prime_2.0 software (Psychology Software Tools, Pittsburgh, PA) was used to present a UCS expectancy rating scale on a video screen (Silent Vision, Avotec, Way Stuart, FL) located above the subject’s head and viewed through a mirror attached to the RF coil. An MR-compatible trackball (Trackball 2, Cambridge Research Systems, Kent, UK) was used to monitor subjects’ expectancy of receiving the UCS. The trackball controlled a rating bar that was presented throughout the conditioning session on the video screen. Subjects were instructed to rate their UCS expectancy from moment to moment using a continuous scale from 0 to 100 (0 = certain the UCS would not be presented, 50 = uncertain whether the UCS would be presented, 100 = certain the UCS would be presented) to reflect their current UCS expectancy. UCS expectancy was calculated as the average response (4 s sample) prior to UCS onset.
Skin Conductance Response
An MR-compatible physiological monitoring system (Biopac Systems; Goleta, CA) was used to collect skin conductance response (SCR) data. SCR was sampled (10 kHz) with a pair of disposable radio-translucent dry electrodes (EL509, Biopac Systems; Goleta, CA). Isotonic recording electrode gel (Gel101, Biopac Systems; Goleta, CA) was applied to the electrodes which were then affixed to the distal phalanx of the index finder and thenar eminence of the non-dominant hand. SCR data were processed using Biopac AcqKnowledge 4.1 software. A 1-Hz low-pass digital filter was applied and SCR data were resampled at 250 Hz. Unconditioned SCRs were calculated by subtracting the skin conductance signal at response onset from the peak skin conductance value during the 10 s immediately following UCS presentation. Data were square root transformed prior to statistical analyses to address violations in normality.
MRI Data
Structural and functional imaging was completed on a 3T MAGNETOM Verio scanner (Siemens Healthcare, Erlangen, Germany). Functional image processing was performed with the Analysis of Functional NeuroImages (AFNI) software package [52]. Echo-planar time series data were despiked, corrected for slice timing offset, motion corrected, aligned, concatenated, reregistered to the fifth volume of the first imaging block, and spatially blurred using a 4-mm full-width-at-half-maximum Gaussian filter. Functional MRI data were analyzed at the individual subject level using the input from all stimuli in a multiple linear regression using a gamma variate hemodynamic response function. Regressors to account for brain activity not related to the UCR included reference waveforms for the CS+, CS−, and head motion parameters. Time points where 3% or more of the voxels across the brain were beyond the voxel-wise trend and median absolute deviation (i.e., outliers) of the time series were censored from the individual subject level analysis. The regressors of interest for this study modeled the unconditioned fMRI signal response to UCS presentations during the CS+UCS and UCS alone. Percent signal change was used as an index of the amplitude of the unconditioned fMRI signal response produced by the UCS. Functional maps reflecting percent signal change were converted to the Talairach and Tournoux stereotaxic coordinate system for group analyses [53].
Data Analysis
Means, standard deviations, frequencies, and percentages were reported for demographic and psychological health data. These data were analyzed using Pearson’s chi-square (χ2) and t-tests. Bonferroni adjustment was made to correct for multiple comparisons. Independent mixed factorial analysis of variance (ANOVA) with group (PTSD vs. DEC) as the between-subjects factor, and condition (predictable vs. unpredictable trials) were used to analyze data from expectancy ratings and skin conductance responses.
Given a priori hypotheses based on prior work [16-18, 23, 54-56], an anatomical mask was used to restrict group level analyses to the prefrontal cortex (PFC), cingulate cortex, inferior parietal lobule, temporal cortex, insula, amygdala, and hippocampus to reduce the number of voxel-wise comparisons. T-test comparisons of the differential response to predictable minus unpredictable threat (i.e., CS+UCS minus UCS alone) were completed to assess group (PTSD versus DEC) differences in threat-elicited brain activity. A voxel-wise threshold of p < 0.005 with activation volumes greater than 300 mm3 (cortical regions) and 40 mm3 (amygdala and hippocampus) resulted in a family-wise error corrected significance threshold of p < 0.05.
RESULTS
Demographics
There was incomplete demographic data on six participants (2 DEC and 4 PTSD group) which resulted in their data being excluded from the analysis. Simple t-test comparisons revealed significant differences between the PTSD and DEC groups on several variables (Table 1). Specifically, the PTSD group was comprised entirely of males, whereas 24% of the DEC group were female. In addition, the PTSD group was less educated, had greater levels of combat exposure, and greater traumatic life events than the DEC group, p < .05. The PTSD group had significantly greater symptoms of posttraumatic stress, depression, anxiety, and daytime sleepiness, p < .05. There were no significant group differences in age, race, childhood family experiences, and alcohol use, p > .05. There was a significant difference in concussion history between the groups, p < .05. None of the participants in the DEC group reported having sustained a concussion within the last eight years compared to 61% (23/38) of the PTSD group. This is not surprising due to the high co-morbidity between concussion and PTSD in military populations [57, 58]. The percentage of medicated participants in the PTSD group was approximately 49% (16/33), which was significantly higher than the 21% (8/38) in the DEC group (χ2 = 5.94, p = .015).
UCS Expectancy
Nine participants (5 DEC and 4 PTSD group) were excluded from the UCS expectancy analysis due to missing or incomplete data (DEC, n = 35; PTSD, n = 34). The mixed factorial ANOVA revealed significant differences in UCS expectancy. There was a significant main effect for predictability (F[1,67] = 51.28, p < .001), but no main effect for group (F [1,67] = 0.02, p = .890) or predictability x group interaction (F [1,67] = 0.20, p = .660). UCS expectancy was greater on CS+UCS trials (66.45 ± 2.42) than on UCS alone (55.69 ± 2.08) trials for the DEC group (t[34] = 4.70, p < .001, d = 4.78). Similarly, the PTSD group also showed greater UCS expectancy on CS+UCS trials (66.75 ± 2.65) than UCS alone (54.57 ± 2.18; t[33] = 5.44, p < .001, d = 4.96) trials.
Skin Conductance Responses
Ten participants (7 DEC and 3 PTSD group) were excluded from the SCR analysis due to missing or incomplete data (DEC, n = 33; PTSD, n = 35). The mixed factorial ANOVA revealed significant learning-related differences in SCR expression to threat (CS+UCS versus UCS alone). There was a main effect for predictability (F [1,66] = 21.87, p < .001) and a main effect for group (F [1,66] = 6.41, p = .012), but no predictability x group interaction (F [1,66] = 1.12, p = .29). Simple t-test comparisons revealed a significantly diminished SCR in response to predictable trials (1.09 ± 0.09) compared to unpredictable trials (1.25 ± 0.09; t[67] = −4.71, p = .001, d = 1.78). Further, the PTSD group showed attenuated SCRs on both predictable (PTSD: 0.89 ± 0.12; DEC: 1.29 ± 0.11; t[66] = 2.44; p = .018, d = 3.47) and unpredictable trials (PTSD: 1.01 ± 0.13; DEC: 1.48 ± 0.12; t[66] = 2.63; p = .011, d = 3.76) compared to the DEC group.
Functional MRI
After inspection of the imaging data, 14 participants (7 DEC and 7 PTSD group) were excluded from the fMRI analyses due to incomplete data and/or excessive movement (DEC, n = 33; PTSD, n = 31). The results indicated there were significant group differences in the differential response between predictable threat and unpredictable threat (i.e., CS+UCS minus UCS alone) within the left amygdala, right hippocampus, right insula, and bilaterally within the superior and middle temporal gyri, p < .05 (Table 2). Group differences in the amygdala and hippocampal response to predictable versus unpredictable threat conditions are illustrated in Fig. (1). There were no significant group differences in the differential response in the PFC, cingulate cortex, or inferior parietal lobe, p > .05.
Region | Vol (mm3) | Talairach coordinates | |||
---|---|---|---|---|---|
x | y | z | t | ||
STG | |||||
Right | 1442 | 58 | −34 | 11 | 4.77 |
Left | 832 | −57 | −20 | 7 | 4.37 |
MTG | |||||
Right | 615 | 42 | −56 | 23 | 4.51 |
Left | 323 | −49 | −31 | 2 | 3.95 |
Insula | |||||
Right | 343 | 40 | -9 | -8 | 4.22 |
Hippocampus | |||||
Right | 86 | 34 | −13 | −15 | 3.69 |
Amygdala | |||||
Left | 42 | −22 | −2 | −14 | 3.40 |
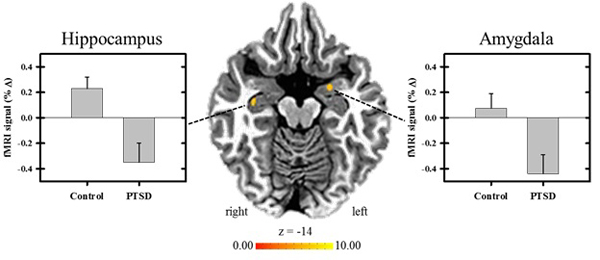
DISCUSSION
In the current study, threat predictability was manipulated by presenting an aversive UCS that was either preceded by a CS (i.e., predictable) or by presenting the UCS alone (i.e., unpredictable). Findings using this approach in healthy individuals have shown diminution of both SCR expression and neural activation to predictable compared to unpredictable threat [16-18, 23, 24]. Both the PTSD and DEC groups showed learning-related changes in threat expectancy and threat-elicited SCR expression. For both groups, threat expectancy ratings were greater to predictable threat than unpredictable threat, and threat-related SCRs were diminished to predictable threat in both groups. However, threat-elicited SCRs were also diminished to unpredictable trials for the PTSD compared to the DEC group. Group differences in the threat-elicited fMRI signal response were observed within the amygdala, hippocampus, insula, and bilateral superior and middle temporal gyri to predictable versus unpredictable threat. Contrary to our hypothesis, the fMRI signal response for the PTSD group was diminished within each of these brain regions during predictable compared to unpredictable threat conditions. In contrast, the DEC group showed increased threat-related activity within each of the brain regions during predictable threat compared to unpredictable threat.
Being that there were no significant differences between the PTSD and DEC groups in threat expectancy, and both groups showed diminished SCRs to predictable threat, group differences in the ability to predict the CS+UCS condition does not explain the direction of the results of the fMRI activation. More specifically, the DEC group’s threat-related neural activation was greater to predictable threat compared to unpredictable threat, which is not consistent with the findings of prior studies [16-18, 23, 24]. In contrast, the PTSD group’s diminished activation of the regions of interest (ROIs) is more in line with what is observed in healthy, non-clinical participants.
Prior work has shown that the hippocampus serves a regulatory role in fear expression [15, 59, 60]. The hippocampus is implicated in the acquisition, extinction, and renewal of fear [61]. Increased threat-elicited hippocampal activity has also been observed in response to unpredictable threat compared to threat that is both predictable and controllable [24]. In addition, our findings revealed diminished insula activation to predictable versus unpredictable threat in the PTSD group. In contrast, the DEC group showed accentuation of insula activation to predictable threat. Linnman et al. [32] showed greater insula activation to predictable threat versus omitted threat in civilians with PTSD compared to controls. Our finding contradicts Linnman et al.’s [32] findings by showing less activation to predictable threat in our PTSD group compared to controls. Yet, based on prior findings [24], the results from our PTSD group are in accord with what would be predicted if these participants were non-clinical controls.
Greater activation of superior and middle temporal regions in response to unpredictable threat was observed in the PTSD group. With the superior temporal gyrus including the auditory cortex, and the middle temporal cortex implicated in auditory expectation [39] with projections to the hippocampus and amygdala, activation of these regions in our PTSD group appears to be moderated by threat predictability.
PTSD has been associated with exaggerated fear responding [32, 33]. However, this appears to be specific to predictable threat. In our study, we provide evidence of hypersensitivity to the unpredictable threat, which might have contributed to the larger differences in ROI activation for the PTSD group compared to the DEC group. Yet, this does not explain why our DEC group had greater ROI activation to predictable threats compared to unpredictable threats.
Prior research suggests that regions within the PFC (e.g., dorsomedial and ventromedial) exert top-down control over the function of other brain regions, such as the amygdala and insula, for regulating emotion responses [34]. In the current study, there were no significant group differences in the fMRI response between predictable versus unpredictable threat in PFC activation. This suggests a similar level of involvement of the PFC between the PTSD and DEC groups, which therefore, did not predict the significant group differences in the other ROIs. Since PFC activation between the groups was not significantly different, we cannot rule out group differences in bottom-up processes in response to the UCS.
One explanation for the DEC group’s lack of diminution across the various ROIs might be altered sensitivity to the UCS in which they perceive the aversive stimulus as less unpleasant than the PTSD group. A major difference from our controls compared to those used in prior imaging studies using the same task was that our participants had prior deployment(s) to Iraq and/or Afghanistan. In deployed service members, those who do not develop PTSD may be subject to neuropsychological changes associated with the deployment environment. Supportive evidence for this was reported by Vasterling and colleagues [62] who compared pre- and post-deployment assessments on 654 Army soldiers. The findings revealed compromised performance on sustained attention, memory, and worse psychological health, but more efficient performance on tests of simple reaction time, none of which were associated with head injury, stress, or depression. All of our controls had prior deployments, and over half were exposed to combat. However, the psychological health scores for the DEC group were within normal ranges, suggesting the absence of clinical and subclinical symptom presentation in our controls. We can only speculate on deployment-related alterations in other neuropsychological functions such as the processing of emotionally valent stimuli, which might have resulted in dampened emotional responsivity to the UCS, and/or accentuated response to predictable threat and attenuated responses to unpredictable threats.
Combat exposure has been linked with lower perceived arousal in healthy, non-clinical soldiers, at least when rating military-related images [63]. Whether this applies to auditory stimuli remains unclear. The fact that the DEC group showed only small differential activation of the amygdala between the predictable versus unpredictable threat conditions potentially supports this interpretation (illustrated in Fig. 1). However, if deployment and/or combat exposure is associated with changes in emotional processing, one would expect to observe abnormal patterns of neural activation in the PTSD group, especially since this group had a significantly greater number of deployments, combat exposure, and psychological health symptoms (i.e., depression, anxiety, daytime sleepiness, and traumatic stress). This explanation is not supported by our findings from the PTSD group’s data and, therefore, only has application to the DEC group. Based on this, it raises the concern about unintentional selection bias when doing cross-sectional studies with clinical populations.
Evidence suggests that a combination of genetic, phenotypic, and environmental factors contribute to vulnerability to developing PTSD. In a longitudinal study by Dretsch and colleagues [64], data from 231 of 458 U.S. Army soldiers were analyzed. The findings revealed that, in addition to combat-related events while deployed, pre-deployment traumatic stress scores and brain-derived neurotropic factor genetic polymorphisms were predictors of post-deployment PTSD. Childhood environment and prior trauma were not significant predictors. Additional studies support the role of individual, pre-trauma differences in predicting PTSD. A recent longitudinal study by Wild and colleagues [65] on 386 of 453 paramedics, found that in addition to a history of mental disorders, premorbid cognitive styles, coping styles, and psychological traits predicted PTSD. Considering these findings, it cannot be ruled out that some of our group differences were mediated by trait-like characteristics representative of a predispositional resilience and/or vulnerability to PTSD.
As an explanation for the findings in the PTSD group, hypersensitivity to unpredictable threat could contribute to accentuated emotional responses to unpredictable threat and attenuated responses when the threat was predictable, resulting in a more robust differential response characterized as diminution to learned threat. Indeed, hypersensitivity to threat seems plausible based on prior findings [36, 37, 66]. Prior findings in healthy individuals, have found that diminution of ROI activation to learned-threat is accompanied by diminution of related SCRs [23, 51]. Since the PTSD group showed a pattern of greater ROI activation to unpredictable threat, one would expect to observe a concomitant pattern of accentuated SCRs. This incongruence in SCRs and ROI activation could be interpreted as some degree of general hypervigilance in the PTSD in anticipation of the unpredictable aversive stimulus.
Other studies have used tactile (shock) [29] and visual (images) [30] stimuli perceived via other sensory modalities as threats. Different threat stimuli could result in variability in the activity of the ROIs selected in the current study, namely parietal and temporal regions. However, it is unlikely that variability in activity of other select ROIs, such as the amygdala, hippocampus, and insula, would occur as a result of sensory modality alone. Rather, difficulties in balancing the level of perceived arousal and valence, and ultimately perceived threat, associated with the stimuli from the different sensory modalities is likely to have a greater effect on specific ROI activation.
One of the limitations in the current study was that both groups contained participants using psychoactive medication. In the PTSD group, the percentage was significantly higher, including the potential for polypharmacy effects. To more thoroughly ascertain the impact of PTSD, future studies should attempt to replicate the procedures with drug-naïve participants in both groups. Researchers should continue to explore the effects of specific pharmacologic interventions on the neurocircuitry associated with various fear conditioning experimental designs in order to provide insight into potential therapeutic effects on PTSD. Another limitation of the study was the lack of granularity concerning co-occurring psychiatric conditions. Although the additional diagnosis of psychotic, bipolar, or personality disorders was an exclusion criterion, there is a high comorbidity of substance use and depression. Because access to the service members’ medical records was for eligibility screening purposes, and not data collection, validated questionnaires were used to assess alcohol use and mood (i.e., depression and anxiety). The PTSD group had significantly greater posttraumatic stress, depression, and anxiety symptoms, but unexpectedly, alcohol use was not significant. Additionally, there was a gender imbalance between the groups in that only the DEC group had female participants. Future studies should explore gender differences with application to PTSD and learned threat.
CONCLUSION
In summary, soldiers with PTSD showed a significantly greater threat-elicited fMRI signal response to unpredictable threat, and diminution to predictable threat, within the amygdala, hippocampus, insula, and superior and middle temporal gyri. As such, the PTSD group’s pattern of neural activation in these regions is somewhat consistent with what has been reported for healthy, non-clinical populations. In contrast, the DEC soldiers showed greater activation of these brain regions to predictable compared to unpredictable threat. This does not appear to be attributed to impaired acquisition in the DEC group in that both groups showed learning-related changes in UCS expectancy and threat-elicited SCR expression. A plausible explanation for atypical findings from our controls is deployment-related neuropsychological changes, which potentially reduced sensitivity to the UCS. In contrast, although the PTSD group appears to be able to regulate their emotional responses to predictable threats, the results might be mediated by hypersensitivity to unpredictable threat in the PTSD group; which may reflect predispositional, trait-like characteristics of vulnerability. As such, this finding may have implications for therapists in terms of individualized cognitive-behavioral treatments. However, successfully addressing this and the other limitations of the study will rely on future longitudinal studies of populations at risk for exposure to psychologically traumatic events.
LIST OF ABBREVIATIONS
AFNI | = Analysis of Functional NeuroImages |
AUDIT | = Alcohol Use Dependency Identification Test |
BTBIS | = Brief Traumatic Brain Injury Screening |
CAPS-5 | = Clinician-Administered PTSD Scale for DSM-5 |
CES | = Combat Exposure Scale |
CFE | = Childhood Family Experiences |
CR | = Conditioned response |
CS | = Conditioned stimulus |
CS+UCS | = Unconditioned stimulus that follows the CS+ |
DEC | = Deployment-exposed controls |
DSM-5 | = Diagnostic and Statistical Manual of Mental Disorders DSM-5 |
ESS | = Epworth Sleepiness Scale |
fMRI | = Functional magnetic resonance imaging |
MRI | = Magnetic resonance imaging |
MRMC IRB | = Medical Research and Materiel Command Institutional Review Board |
PCL-5 | = PTSD Checklist |
PFC | = Prefrontal cortex |
PTSD | = Posttraumatic stress disorder |
ROIs | = Regions of interest |
SCR | = Skin conductance response |
TBI | = Traumatic brain injury |
UCR | = Unconditioned response |
UCS | = Unconditioned stimulus |
UCS alone | = Unconditioned stimulus presented alone |
ZDS | = Zung Depression Scale |
ZAS | = Zung Anxiety Scale |
APPENDIX A
PTSD Screening. The Clinician-Administered PTSD Scale (CAPS-5; Weathers et al., 2013) was used to evaluate PTSD symptoms. This 30-item structured interview is the “gold standard” in PTSD assessment and is used to make the PTSD diagnosis. CAPS-5 combines information about frequency and intensity of an item into a single severity rating. A total score is calculated by summing severity scores for the 20 DSM-5 PTSD symptoms. Similarly, CAPS-5 symptom cluster severity scores are calculated by summing the individual item severity scores for symptoms corresponding to a given DSM-5 cluster: Criterion B (items 1-5); Criterion C (items 6-7); Criterion D (items 8-14); and Criterion E (items 15-20). PTSD is determined by first dichotomizing each symptom as “present” (a score of 2 for moderate or higher) or “absent,” then applying the DSM-5 diagnostic rule which requires: At least one Criterion B symptom; at least one Criterion C symptom; at least two Criterion D symptoms; at least two Criterion E symptoms; Criterion F is met (disturbance has lasted one month); and Criterion G is met (disturbance causes either clinically significant distress or functional impairment).
PTSD Checklist-5 (PCL-5; Weathers et al., 2013). The PCL-5 is a 20-item self-report measure that assesses the 20 DSM-5 symptoms of PTSD. The PCL-5 has a variety of purposes, including screening individuals for PTSD, making provisional PTSD diagnoses, and monitoring symptom change during and after treatment. Items are rated using a 5-point likert scale; 0 = “Not at all” to 4 = “Extremely.” A total symptom severity score (range: 0-80) can be obtained by summing the scores for each of the 20 items with a cut score of 38 for a provisional diagnosis of PTSD.
Combat Exposure Scale (CES; Guyker et al., 2013). The CES is a 7-item self-report measure that assesses wartime stressors experienced by combatants. Items are rated on a 5-point frequency, 5-point duration, 4-point frequency, or 4-point scale. A total exposure score ranging from 0 to 41 is calculated.
Childhood Family Experiences (CFE; King, King, & Vogt, 2003). The CFE is one in a set of 13 subscales from the Deployment Risk and Resilience Inventory (DRRI; King et al., 2003) that evaluate a number of risk and recovery factors during different stages of deployment that might affect an individual’s development of stress-related illness. A total score ranging from 12 to 60 is calculated by summing the scores for each of the 12 items. Higher scores reflect positive experiences.
Zung Depression Scale (ZDS; Zung, 1971a). The ZDS is a 20-item scale measuring self-reported symptoms of depression. The ZDS produces scores ranging from 20 through 80; 20 – 44 for normal range, 45 – 59 for mildly depressed, 60 – 69 for moderately depressed, and 70 and above for severely depressed.
Zung Anxiety Scale (ZAS; Zung, 1971b). This ZAS is a 20-item, self-report anxiety rating scale. The ZAS has scores ranging from 20 through 80; 20 – 44 for normal range, 45 – 59 for mild to moderate anxiety, 60 – 74 for marked to severe anxiety, and 75 – 80 for extreme anxiety.
Alcohol Use Dependency Identification Test (AUDIT; Saunders, Aasland, Babor, De la Fuente, & Grant, 1993). The 10-item instrument is used to assess alcohol use. A score of 8 or more in men, and 7 or more in women, indicates likelihood of hazardous or harmful alcohol use. A score of 20 or more suggests potential alcohol dependence. A total score ranging from 0 to 40 is calculated by summing the scores for each of the items.
Epworth Sleepiness Scale (ESS; Johns, 1991). This instrument asks the subject to rate his or her probability of falling asleep on a scale of increasing probability from 0 to 3 in eight different situations. A total sleepiness score ranging from 0 to 24 is calculated by summing the scores for each of the items.
Brief Traumatic Brain Injury Screen (BTBIS; Schwab et al., 2006). This three-item assessment is used by the Army for identifying soldiers that may have sustained a prior mild TBI or concussion. Participants were asked to report if they had sustained a concussion from childhood to the present based on a diagnosis from a medical professional or meeting the criteria of the BTBIS. A positive concussion score requires both the endorsement of an injury-related event, and at a minimum, an altered state of consciousness (e.g., being dazed, confused, or seeing stars; posttraumatic amnesia; loss of consciousness < 20 minutes).
CONFLICT OF INTEREST
The authors confirm that this article content has no conflict of interest.
ACKNOWLEDGEMENTS
This project was funded by the U.S. Army Medical Research and Materiel Command (USAMRMC), Military Operational Medicine Research Program, and was supported in part by an appointment to the Internship/Research Participation Program for the USAMRMC, administered by the Oak Ridge Institute for Science and Education (ORISE) through an agreement between the U.S. Department of Energy and the USAMRMC. We would like to thank the staff and leadership at the USAARL and Auburn University for their support that made this study possible. Dr. Dretsch was the principle investigator (PI) of the study who secured funding, developed the protocol, analyzed the psychological health data, and was the primary author of the manuscript. Dr. Wood and Dr. Knight contributed to the study’s development and provided oversight to the analysis of MRI and SCR data. Ms. Wheelock and Dr. Wood were involved with analyzing the MRI and SCR data. Dr. Daniel, Dr. Jeff Katz, Dr. Goodman, and Dr. Deshpande collected data and assisted with analysis of MRI data. Dr. Denney was the site PI who provided oversight to data collection. Dr. Traynham assisted with recruitment and enrollment of participants.