Long-Term (Six Years) Clinical Outcome Discrimination of Patients in the Vegetative State Could be Achieved Based on the Operational Architectonics EEG Analysis: A Pilot Feasibility Study
Abstract
Electroencephalogram (EEG) recordings are increasingly used to evaluate patients with disorders of consciousness (DOC) or assess their prognosis outcome in the short-term perspective. However, there is a lack of information concerning the effectiveness of EEG in classifying long-term (many years) outcome in chronic DOC patients. Here we tested whether EEG operational architectonics parameters (geared towards consciousness phenomenon detection rather than neurophysiological processes) could be useful for distinguishing a very long-term (6 years) clinical outcome of DOC patients whose EEGs were registered within 3 months post-injury. The obtained results suggest that EEG recorded at third month after sustaining brain damage, may contain useful information on the long-term outcome of patients in vegetative state: it could discriminate patients who remain in a persistent vegetative state from patients who reach a minimally conscious state or even recover a full consciousness in a long-term perspective (6 years) post-injury. These findings, if confirmed in further studies, may be pivotal for long-term planning of clinical care, rehabilitative programs, medical-legal decisions concerning the patients, and policy makers.
INTRODUCTION
Patients with severe brain damage have different clinical outcomes that usually manifest within a year after injury [1-3]. The worst possible outcome of acquired brain injury (excluding death) is a vegetative state, recently named “unresponsive wakefulness syndrome” (VS/UWS) [4]. The VS/UWS is commonly agreed to be a state of “wakeful unconsciousness” [1] of self and environment in which “the patient breathes spontaneously, has a stable circulation, and shows cycles of eye closure and opening which may simulate sleep and waking” [5]. Another outcome or stage of recovery is characterised by a minimally conscious state (MCS), which is “a condition of severely altered consciousness in which minimal but definite behavioural evidence of self or environmental awareness is demonstrated” [6]. MCS often fluctuates, especially at the borderline with VS/UWS, meaning that it transiently appears and disappears. Finally, emergence from MCS (E-MCS) is defined by the return of full conscious communication and/or object use [6] and is consistent with full awareness of self, others, and the environment [7].
Currently electroencephalogram (EEG) recordings are increasingly used to evaluate patients with disorders of consciousness (DOC) [8]; and accumulated published data indicate the importance of EEG when assessingthe degree/type of brain damage and the prognosis of patients in the early stages of DOC [9-14]. The EEG analysis approaches include either the simplest conventional measures based on the qualitative visual evaluation of EEG traces or the use of ad hoc scales [10, 11, 15], or a number of analytical algorithms aiming at extracting quantitative EEG parameters in an attempt to classify the severity of brain damage or assess outcome prognosis [16-25]. EEG is a particularly well-suited technology for this purpose: it is routinely available in most clinics/laboratories, non-invasive, and relatively inexpensive, it allows for repeated or extended measurements at the bedside, and it directly measures neuronal dynamics that is remarkably correlated with behavior, cognition, and consciousness [26-31].
However, there is a lack of information concerning the effectiveness of EEG analysis for the ascertainment of long-term (many years) outcomes in chronic DOC patients [32], despite the fact that it may have crucial importance for long-term planning of clinical care, rehabilitative programs aiming to improve the patients’ wellbeing and recovery, and medical-legal decisions concerning the patients, as well as for the families of patients and policy makers. For example, since 90s Dutch ethical, medical, and legal legislation considers life-sustaining treatment, including artificial nutrition and hydration (ANH), for the sole purpose of prolonging VS/UWS beyond reasonable chances of recovery of consciousness to be medically futile [33] and a violation of human dignity [34].
Hence, the aim of the present retrospective study was to use the 6-year post-injury assessment of long-term DOC patient outcomes (VS/UWS, MCS, E-MCS) in order to find the discriminating parameters in the EEG data recorded within 3 month post-injury. EEG measures that are based on the theory of consciousness are particularly attractive for this purpose1 because they have critical practical implications in the clinic [40], allowing indexing and distinguishing unconscious states from the presence of minimal or full consciousness [14, 43]. Operational architectonics (OA) methodology for EEG analysis [28, 30, 44, 45] is one of such approaches.
A comprehensive review of this methodology can be found elsewhere [31, 46]. Here we provide only a brief summary. In short, according to the OA theory [45] local fields of transient functional neuronal assemblies are equivalent to operations that can be conscious (phenomenal2). For example, some neuronal assemblies display preferential processing for color, others for shape, yet others for motion, smell, etc. [48]. Such simple operations responsible for qualia are reflected in the electrical brain field (EEG) in the form of local quasi-stationary segments that can be conceptualized as standing waves within a 3D volume (for a review see [31, 44-46]), and could be measured using adaptive EEG segmentation procedure [31, 46]. It has been experimentally shown that parameters of EEG segments (number, duration, amplitude, and others) are reliably and consistently correlated with changes in the phenomenal (subjective) content both during spontaneous (stimulus independent) and induced (stimulus dependent) experimental conditions [31]. At the same time, to have a phenomenal experience of any complex object/concept or scene, several simple features of that object/concept/scene should be spatially and temporally integrated [45]. According to OA theory, this complexity requires temporally coordinated operations (equivalent of synchrony of local bioelectrical fields) of many neural assemblies, which selectively emerge from the entire brain (for a detail discussion see [45]). This process can be measured directly using an EEG operational synchrony index [31, 46]. Several synchronized complexes of neuronal assemblies (so-called operational modules - OMs) can further synchronize among each other forming even more complex spatial-temporal structures, thus constituting a clear nested functional hierarchy [49] allowing a conscious mind to be expressed [29, 30, 45]. This OA strategy of EEG analysis has been validated in a number of electrophysiological, cognitive, and clinical studies and is proven to be robust, consistent, and statistically reliable (for a review see [29-31, 44-46]).
OA analysis of DOC patients’ EEGs revealed that the absence of consciousness in patients in VS/UWS is paralleled by dramatic impairment of overall EEG operational architecture (Fig. 1): neuronal assemblies diminish, their life span shortens significantly, and they become highly unstable and functionally disconnected (desynchronized) from one another, including the default mode network [21, 29]. At the same time, fluctuating (minimal) awareness in patients in MCS was paralleled by partial restoration of EEG operational architecture: increased size, life span, and stability of neuronal assemblies, together with an increased number and strength of functional connections among them (Fig. 1),approaching the level found in healthy fully conscious participants [21, 29]. Foremost, OA methodology was able to reliably discriminate (at the group level) between patients in VS/UWS and MCS independent of brain damage etiology, thus indicating that OA EEG parameters capture consciousness phenomenon itself rather than physiological parameters of brain damage [35]. Further, OA EEG parameters registered 3 months post-injury (in patients in VS/UWS) were able to reliably predict consciousness recovery 6 months post-injury [23].
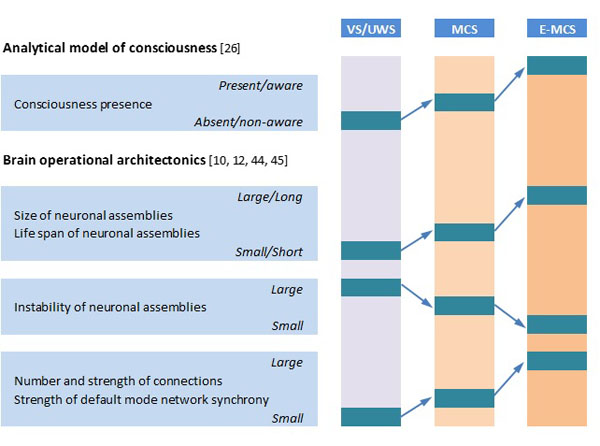
Schematic presentation of relation of analytical model of consciousness to brain (EEG) operational architectonics parameters. Abbreviations: EEG: electroencephalogram; VS/UWS: vegetative state/unresponsive wakefulness syndrome; MCS: minimally conscious state; E-MCS: emergence from minimally conscious state to full consciousness. Further explanations are provided in the text.
In light of these results, it is reasonable to test whether EEG operational architectonics parameters could also be useful in determining very long-term (6 years) clinical outcomes of patients in VS/UWS whose EEGs were registered within 3 months post-injury.
MATERIALS AND METHODOLOGY
Patient Cohort
For the purpose of this pilot feasibility study, based on clinical evaluations at six years follow-up after the 3 month post-injury EEG registration, patients with traumatic brain injury who were admitted in Neurorehabilitation Unit at the Fondazione Istituto “San Raffaele - G. Giglio” and met the accepted international definition of VS/UWS [5, 50], were retrospectively divided into three groups: unrecovered - continued to be vegetative (VS-Pers), recovered minimally conscious state (VS-MCS), and recovered full consciousness - exit from MCS (VS-E-MCS). Patients’ demographic information is summarized in the Table 1. On the day of EEG registration (about 3 months post-injury) the Levels of Cognitive Functioning (LCF) scale score [51] was assessed to estimate the expression of clinical consciousness [10, 11]. The LCF has a linearly graded scale ranging from 1 to 8 (1 - patient is unconscious; 8 - patient is self-oriented and conscious of the environment) and is well correlated with resting-state EEG abnormalities in patients with brain damage [10, 11]. At the time of EEG scanning (about 3 months post-injury), all patients had an LCF score of 1 or 2 (1.3±0.6). Current LCF scores (6 years post-injury) are presented in the Table 1.
Inclusion criteria for the patients to be recruited for this study were (a) being alive 6 years post-injury, (b) confirmation of diagnosis of VS/UWS, MCS or E-MCS according to the diagnostic criteria [5, 50], (c) first-ever acute brain event; (d) stable LCF score during 3 days after admission and six years later. Exclusion criteria comprised (a) death and (b) inconclusive LCF score. These inclusion/exclusion criteria resulted in the recruitment of three patients (from the pool of fourteen VS/UWS patients): one VS-Pers patient (age = 35 years), one VS-MCS patient (age = 55 years), and one VS-E-MCS patient (age = 19 years).
The evaluations and manipulations (EEG recording) used for the purpose of this study did not interfere with the usual medical practice, or with the everyday rehabilitation therapies and were part of a routine screening of severe brain-injured subjects during their rehabilitation program. The study was approved by the local institutional Ethics Committee and complies with Good Medical Practice. Informed and overt consent by legal representatives of the patients was obtained, in line with the Code of Ethics of the World Medical Association (Declaration of Helsinki) and standards established by the Fondazione Istituto “San Raffaele - G. Giglio” Review Board. Data use was authorized by means of written informed consent of the VS/UWS patients’ caregivers.
Basic demographic and clinical characteristics of patients.
![]() |
EEG Registration
Waking resting EEG was recorded with a Neuropack (Nihon Kohden, Japan) from 19 electrodes positioned according to the International 10-20 system. The recording parameters were: 0.5-70 Hz bandpass; 200 Hz sampling rate; ~30 min. The impedance was below 5-10 kΩ. An electrooculogram (0.5-70 Hz bandpass) was also collected. Cephalic EEG reference (mean of the signals from C3 and C4 electrodes) was used. Even though there are propositions that the cephalic reference may result in an under- or over-estimation of the potentials, as we have discussed in [29] the amplitude variability (envelope) in the delta, theta, alpha, and beta bands did not vary significantly as a function of reference or measurement electrode impedance and that at present no agreement on a preferred solution to the reference issue is established [29].
EEG recordings were started if patients had their eyes open spontaneously, the eyelids were then closed by hand and kept closed until the end of registration. At the end of the recordings all patients opened their eyes spontaneously, suggesting an unchanged vigilance level throughout EEG registration. The presence of an adequate EEG-signal was determined by visual inspection of the raw signal. Epochs containing artifacts due to eye movement, eyes opening, significant muscle activity, and movements on EEG channels were marked and then automatically excluded from further analysis.
For each patient an artefact-free EEG stream was fragmented into consecutive 1-minute epochs. Further data processing was performed on each separate 1-minute epoch. Prior to OA analysis, each 1-minute epoch was bandpass-filtered (Butterworth filter of the sixth order) in the alpha (7-13 Hz), beta-1 (15-25 Hz) and beta-2 (25-30 Hz) frequency bands. Phase shifts were eliminated by forward and backward filtering. The mentioned frequency bands were chosen based on our previous studies involving DOC patients [21, 23, 29, 35] due to a fact that only these frequency oscillations have shown dynamics consistent with the analytical consciousness model [20]. According to this model (Fig. 1), OA EEG features responsible for the subjective (un)awareness of self and environment should satisfy one of the following rules: (1) NORM > MCS > VS/UWS (for awareness) or (2) NORM < MCS < VS/UWS (for unawareness) [20].
EEG Segmentation: Estimation of the Neuronal Assemblies’ Attributes
In short, the adaptive EEG segmentation algorithm can be described in two main stages (see for details [31, 46]): (1) preliminary identification of the boundaries between quasi-stationary segments using automated algorithm that moves a double window screening along each separate EEG channel; (2) selection of actual (real) boundaries based on the steepness of previously detected EEG amplitude changes and Student criteria. Three key EEG segment attributes were further estimated: (1) average amplitude within each segment (microvolts) - informs about the size of neuronal assemblies; (2) average length of segments (milliseconds) - informs about life-span of neuronal assemblies; (3) coefficient of amplitude variability within segments (%) - informs about instability of neuronal assemblies [26, 28, 31, 46].
Synchronisation of EEG Segments among EEG Channels: Estimation of the Remote Operational (Functional) Synchrony
Index of Operational Synchrony (for details see [31, 46]) estimates synchronization of EEG quasi-stationary segments obtained from different brain locations. In brief, each boundary in one EEG channel (from any pair of EEG channels) was surrounded by a short (ms) “window”. Any boundary from another channel was considered to coincide if it fell within this window. To arrive at a direct estimate of statistical significance (P < 0.05) of the index, computer simulation of boundaries coupling was undertaken based on random shuffling of segments (500 independent trials) for each pair of EEG channels [31, 46]. As a result of this procedure, the stochastic levels of boundaries coupling, together with the upper and lower thresholds of significance (P < 0.05) were calculated. If there is no synchronization, the index tends toward zero, whereas positive (above upper stochastic threshold) or negative (below lower stochastic threshold) values are indicative of synchronization (coupling of EEG segments is observed significantly more often than expected by chance as a result of random shuffling during a computer simulation) or de-synchronization (coupling of EEG segments is observed significantly less than expected by chance as a result of random shuffling during a computer simulation) respectively [31, 46].The strength of EEG operational synchrony is proportional to the actual value of the index in each pair of EEG channels: the higher this value, the greater the strength of functional connection among neuronal assemblies located in different cortical locations [31]. The number of connections among neuronal assemblies corresponds to the number of statistically valid synchronous pairs of EEG channels [31] and is presented as a percent from the maximum possible number of connections among 19 EEG electrodes.
Statistics
For each analysed state (VS-Pers, VS-MCS, and VS-E-MCS), averages and respective standard deviations were calculated for each of the EEG operational architectonics parameters (size of neuronal assemblies, life-span of neuronal assemblies, instability of neuronal assemblies, number and strength of functional connections among neuronal assemblies) for the whole pull of corresponding 1-minute EEGs (n of 1-minute EEGs for VS-Pers = 20, n for VS-MCS = 26, n for VS-E-MCS = 19). Robust linear regression analyses were performed across three states (VS-Pers, VS-MCS, and VS-E-MCS) for each OA EEG parameter to test for the relation (trend estimation) between changes observed across states of consciousness 6 years post-injury and studied EEG parameters 3 months post-injury. Such linear regression analysis that estimates a “trend line” is often used to argue that a particular parameter caused observed changes at a particular point in time [52]. R-squared (R2) - indicates the square of the residuals of the data after the fit. R2 value of 0.8-0.9 indicates a nearly perfect fit of the prediction to the data, and R2 value of 1 indicates a perfect fit. From a purely statistical point of view, it is feasible to estimate the regression line with as few as 3 estimates if data change is not random [52]; therefore the three states in our study (VS-Pers, VS-MCS, and VS-E-MCS) are sufficient for this analysis.
RESULTS
Features and Dynamics of Neuronal Assemblies (Measured by EEG Segmentation)
Fig. (2) presents the mean values and result of linear regression analysis for OA EEG segment attributes that characterize different features of neuronal assemblies for all EEG locations and three states of consciousness (VS-Pers, VS-MCS and VS-E-MCS). Corresponding data are presented separately for 3 features of neuronal assemblies (size of neuronal assemblies, life-span of neuronal assemblies, instability of neuronal assemblies). One can see, that all studied parameters followed identical relations among VS-Pers, VS-MCS and VS-E-MCS sates: a linear increase in “size” (R2 = 0.95–0.99 for different frequency bands) and “life-span” (R2 = 0.88–0.99 for different frequency bands), and decrease in “instability” (R2 = 0.87–0.97 for different frequency bands) from VS/UWS state (VS-Pers) to minimally conscious state (VS-MCS) and further to the emergence from minimally conscious state (VS-E-MCS) 6 years post-injury (Fig. 2). This observation was similar for all 3 frequency bands (alpha, beta-1, and beta-2).
Operational Synchrony of Neuronal Assemblies (Measured by EEG Segments Synchronization)
Fig. (3) presents the mean values and result of linear regression analysis for number and strength of functional connections for all EEG pair combinations that characterize remote functional connectivity between neuronal assemblies for three states of consciousness (VS-Pers, VS-MCS and VS-E-MCS). Corresponding data are organized the same way as in Fig. (2). We observed that both studied parameters followed identical relations among VS-Pers, VS-MCS and VS-E-MCS sates: a linear increase in “number” (R2 = 0.84–0.95 for different frequency bands) and “strength” (R2 = 0.95–0.96 for different frequency bands) of functional connections from VS/UWS state (VS-Pers) to minimally conscious state (VS-MCS) and further to the emergence from minimally conscious state (VS-E-MCS) 6 years post-injury (Fig. 3). A similar direction of differences was observed in all 3 (alpha, beta-1, and beta-2) frequency bands.
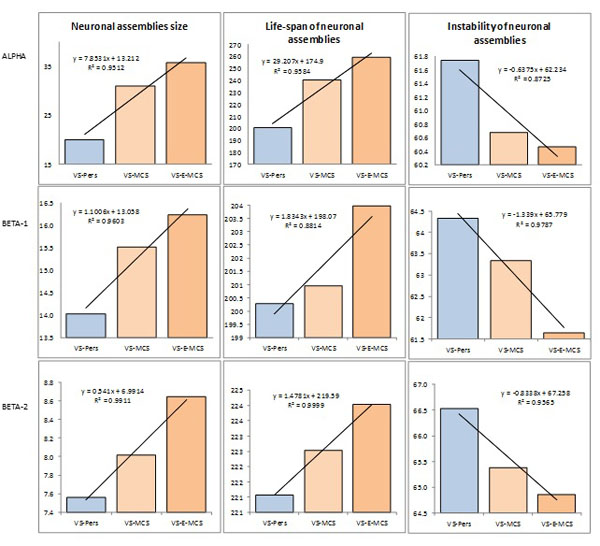
Features and dynamics of neuronal assemblies (indexed by EEG segment attributes) as a function of clinical outcome 6 years post-injury: Linear regression analysis. Data are averaged across all EEG channels and all 1-minute EEGs within each state. The values of attributes of neuronal assemblies indicated by the Y-axis: size of neuronal assemblies-amplitude within each segment (microvolt); life-span of neuronal assemblies-length of segments (milliseconds); instability of neuronal assemblies-coefficient of amplitude variability within segments (%). Lines in the graphs represent the linear regression equation and R2. Abbreviations: Alpha: EEG rhythm 7-13 Hz; Beta-1: EEG rhythm 15-25 Hz; Beta-2: EEG rhythm 25-30 Hz. EEG: electroencephalogram; VS-Pers: patient continues to be in persistent vegetative state 6 years post-injury; VS-MCS: patient continues to be in minimally conscious state 6 years post-injury; VS-E-MCS: patient emerges from minimally conscious state 6 years post-injury.
DISCUSSION
To the best of our knowledge, this is the first study of EEG parameters that has been registered in patients who meet all clinical criteria for the VS/UWS [5, 28] within 3 months post-injury and that could discriminate a very long-term (6 years) clinical outcomes of these patients. Here we have demonstrated that operational architectonics parameters of EEG structure have the capacity to discriminate patient in VS/UWS who continues to be in persistent VS/UWS from those who continue to be in MCS or even emerge from MCS (E-MCS) in a long-term perspective (6 years). More specifically, the larger the neuronal assemblies size, life-span, stability and functional connectedness among neuronal assemblies, the more likely a VS/UWS patient to partially or totally regain consciousness in a long-term perspective (Figs. 1, 2). These results are in line with an emerging consensus regarding modern understanding of the basic requirement for consciousness - the intact dynamic binding of operations performed by multiple neuronal assemblies, which are organized within a nested hierarchical brain architecture [16, 28-30, 53, 54]. In this context the state of unconsciousness would be characterized by brain cortical activity which is broken down into causally independent, small, and short-lived functional modules producing local responses not capable of supporting the subjective content [35, 41, 55] - which is exactly what we observed in persistent VS/UWS state (Figs. 1, 2). However, if these different parameters of brain operational architecture cross a threshold for the minimal neuronal mechanisms that are jointly sufficient for any single specific conscious content - the critical level at which awareness of the environment and of the self can be reliably supported and self-regulated by the brain, then the patient is likely to regain consciousness [43].
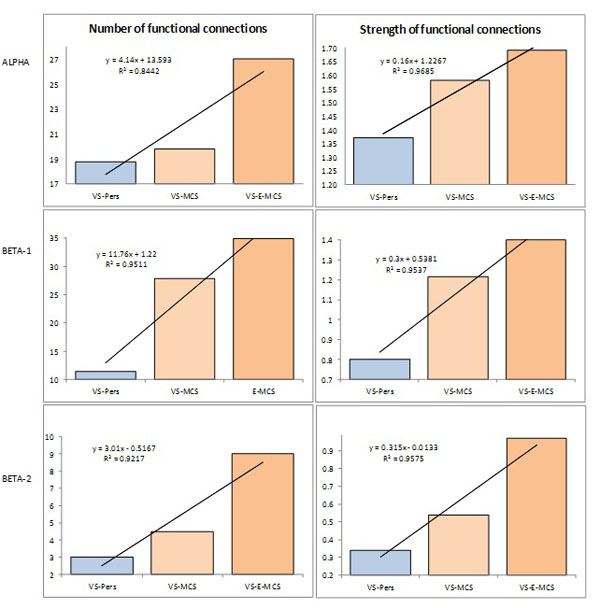
Operational synchrony among neuronal assemblies (indexed by EEG segments synchronization) as a function of clinical outcome 6 years post-injury: Linear regression analysis. Data are averaged across all pairs of EEG channels and all 1-minute EEGs within each state. The Y-axis presents values of either number (%) or strength of functional connections. Lines in the graphs represent the linear regression equation and R2. Abbreviations: Alpha: EEG rhythm 7-13 Hz; Beta-1: EEG rhythm 15-25 Hz; Beta-2: EEG rhythm 25-30 Hz. EEG: electroencephalogram; VS-Pers: patient continues to be in persistent vegetative state 6 years post-injury; VS-MCS: patient continues to be in minimally conscious state 6 years post-injury; VS-E-MCS: patient emerges from minimally conscious state 6 years post-injury.
Further, similar effects across alpha and beta frequency bands observed in this study (Figs. 1, 2) extend several lines of evidence on the strong implication of cortical alpha and beta rhythms for human higher functions and consciousness [20, 29, 56, 57]. This interpretation is in line with current views that conscious awareness might depend on the dynamic formation-disassembling of neuronal assemblies synchronized on different temporal scales across various frequency bands [58-61].
It is important to keep in mind that although patients (who six years after brain-injury reached MCS or even E-MCS condition) have demonstrated evidence of EEG operational architecture closer to actual patients in MCS or normal fully conscious subjects, they all were in VS/UWS condition during the EEG registration and their EEG operational architecture parameters did not differ from the analogues parameters estimated on the group level of patients in VS/UWS [29].
To conclude, the results of the present study suggest that EEG recorded at third month following brain damage, when reliable communication with patients could not yet be established and before spontaneous EEG showed significant modifications in the conventional (based on spectral analysis) parameters (see Table 1), if analyzed by the methods that tailored towards detecting parameters of consciousness rather than to neurophysiological processes [35-42], contains potentially useful information on the outcome of persistent VS/UWS patients 6 years later.
However, despite such promising findings, the very low number of investigated patients (one patient for each state: VS/UWS, MCS, E-MCS) suggests that evaluation of the results should be done with caution before providing indications for clinicians and should be considered preliminary. To confirm the results presented in this article, future prospective studies with a larger group of patients for each state are warranted. Nevertheless, the preliminary results of the present study are strengthened by the fact that the characterizations of the states (VS/UWS, MCS and E-MCS) in terms of EEG operational architectonics revealed in the present study coincide precisely with our previous EEG studies of DOC patients [21, 23, 29, 35]. Moreover, in trend analysis (linear regression) that has been used in the present study as a main statistical method, the observations (or units of analysis) relate to time periods (years, months, days) and not to individuals [62].
Another possible interpretation of the results may relate to the age and the extent of the brain damage (see Table 1). Even though such interpretation may have sense for the youngest patient with the smallest brain damage who recovered full consciousness, it fails with the patient who continued to “stay” in the VS/UWS and who had the intermediate age and brain damage when compared with MCS patient and E-MCS patient (Table 1). Therefore, it is unlikely that age and extend of brain damage could explain the results of the current study. Furthermore, in [29] it has been argued with the examples, that loss of consciousness most likely is related with functional alterations in cortical structures and impairment in relations between them, rather than with particular brain lesions and the amount of brain damage. However, to confirm the presented results in this paper, future studies that include a larger group of patients is warranted.
ABBREVIATIONS
FUNDING
The authors received no financial support or funding for the research, authorship, and/or publication of this article.
CONFLICT OF INTEREST
The authors confirm that this article content has no conflict of interest.
ACKNOWLEDGEMENTS
The authors thank C. Prestandrea (neurophysiology technician) for EEG recordings; C. Neves (Computer Science specialist) for programming, technical, and IT support; D. Skarin for English editing.