Sex Differences in Gamma Band Functional Connectivity Between the Frontal Lobe and Cortical Areas During an Auditory Oddball Task, as Revealed by Imaginary Coherence Assessment
Abstract
We studied sex-related differences in gamma oscillation during an auditory oddball task, using magnetoencephalography and electroencephalography assessment of imaginary coherence (IC). We obtained a statistical source map of event-related desynchronization (ERD) / event-related synchronization (ERS), and compared females and males regarding ERD / ERS. Based on the results, we chose respectively seed regions for IC determinations in low (30-50 Hz), mid (50-100 Hz) and high gamma (100-150 Hz) bands. In males, ERD was increased in the left posterior cingulate cortex (CGp) at 500 ms in the low gamma band, and in the right caudal anterior cingulate cortex (cACC) at 125 ms in the mid-gamma band. ERS was increased in the left rostral anterior cingulate cortex (rACC) at 375 ms in the high gamma band. We chose the CGp, cACC and rACC as seeds, and examined IC between the seed and certain target regions using the IC map. IC changes depended on the height of the gamma frequency and the time window in the gamma band. Although IC in the mid and high gamma bands did not show sex-specific differences, IC at 30-50 Hz in males was increased between the left rACC and the frontal, orbitofrontal, inferior temporal and fusiform target regions. Increased IC in males suggested that males may acomplish the task constructively, analysingly, emotionally, and by perfoming analysis, and that information processing was more complicated in the cortico-cortical circuit. On the other hand, females showed few differences in IC. Females planned the task with general attention and economical well-balanced processing, which was explained by the higher overall functional cortical connectivity. CGp, cACC and rACC were involved in sex differences in information processing and were likely related to differences in neuroanatomy, hormones and neurotransmitter systems.
INTRODUCTION
Recent developments in neuroimaging using magnetoencephalography (MEG) and electroencephalography (EEG) have revealed complex structural and functional organization in brain networks. Event-related potential (ERP) studies show that cognitive and executive functions are closely related to coordinated activity of large-scale networks in the brain.
We studied sex differences in neuro-cognitive processing and showed differences in functional organization cognitive tasks using EEG and MEG. The P300 component is most commonly elicited using the oddball paradigm in clinical studies, and reflects an information-processing cascade when attentional and memory mechanisms are engaged. P300 is sensitive to psychological variables, is linked to attention and memory following local stimuli and is modulated by excitatory and inhibitory mechanisms [1]. In addition, P300 is associated with a circuit pathway between frontal and temporal/parietal brain areas [2].
Sex-related differences in P300 have been intensively investigated and reported regarding emotion and cognition [3-10]. On the other hand, only a small number of studies have examined sex differences in the gamma band using the oddball task. One study showed a time related increase in brain activity in the gamma band in males whereas females displayed a reverse pattern for this relationship [11]. Sex differences have been investigated in patients with schizophrenia, a healthy male group displayed a pattern of earlier gamma (40 Hz) latency in the right compared with the left hemisphere, while the female healthy group displayed a delayed gamma peak frontally compared with the posterior region [12], and female patients with schizophrenia showed additional breakdown in brain network connectivity which may develop with illness chronicity [13]. These reports suggested the importance of investigations of sex differences related to the functional neuronal network in the gamma band in not only normal subjects but also schizophrenic patients.
Neuronal gamma-band synchronization can be induced in many cortical areas by different stimuli or tasks, and is related to several cognitive capacities. Gamma activity plays a key role in neuronal communication, synaptic plasticity, and central cognitive functions, including perceptual biding, attentional selection and working memory maintenance [14, 15]. Activated neural networks are engaged in synchronous rhythmic activity in the gamma frequency range, and the gamma band synchronization affects neuronal interaction [16]. Synchronized firing between cells in different functional columns is associated with rhythmic discharge in the gamma band (20 - 70 Hz), suggesting a functional link between gamma oscillations and response synchronization [17]. Gamma-band synchronization promotes the transfer of relevant information between distinct brain systems [18], and represents reference signals for temporal coding, sensory binding of features into a coherent percept, and storage and recall of information [19]. Specific gamma band (60-80 Hz) activity is critically implicated in the maintenance of relevant working memory information in the prefrontal cortex [20]. Early gamma-band responses were assumed to be related to the match between bottom-up and top-down information, and that late gamma-band activity was assumed to reflect the readout and utilization of the information resulting from this match [21]. Mid-gamma band activity is closely related to cognitive and executive functions, such as binding, polymodal integration, working and long-term memory, motor-planning, and access to consciousness [14, 22], while high frequency activity was modulated by spatial attention in response to both consciously seen and unseen stimuli [23].
Coherence has frequency specificity and transient nature of interactions between signals of sensors covering different scalp areas [24]. The coherence hypothesis (communication-through-coherence [CTC] hypothesis) states that functional connectivity is mechanistically subserved by neuronal coherence, and activated neuronal groups oscillate and thus undergo rhythmic excitability fluctuations that produced temporal windows for communication [25]. Increased coherence between two brain areas shows that this phase difference tends to remain constant, and the relationship between the signals generated may be well approximated by a linear transformation; however this does not necessarily imply that the underlying neocortical dynamics are linear [26]. For neuronal groups within one cortical area, coherent oscillations are mostly found in the gamma-frequency range, between 30 and 100 Hz.
In this study, we examined sex differences in event-related desynchronization (ERD) and event-related synchronization (ERS) in the gamma band (low: 30 - 50 Hz, mid: 50 - 100 Hz, high: 100 - 150 Hz) during an oddball task. Stimulus-induced power modulation of brain activity is referred to as ERD and ERS. ERD is defined as a proportional power decrease, and ERS is defined as a proportional power increase in relation to the baseline activity determined from a specific reference interval which is usually placed several seconds before trigger onset [27-30]. ERD/ERS provides useful insight into brain information [31].
We hypothesized that if gender differences in ERD/ERS in the gamma bands are observed in certain regions, these differences will be related to changes in coherence between these certain regions (seeds) and other cortical regions. Coherence reflects synchronization between two signals based mainly on phase consistency, and high coherence occurs when this phase difference tends to remain constant. MEG topographically shows more focal gamma activity than did the EEG [32], and we thus employed MEG to investigate neuronal coherence. Based on areas with sex differences in ERD/ERS, we calculated imaginary coherence (IC) between seeds and other brain regions.
METHODS
Subjects
We examined 15 males (mean age 27.9±4.00 years) and 15 females (mean age 25.8±5.59 years); there was no significant difference in age present between the two groups (Table 1). All subjects were right-handed, healthy workers in Fujimoto General Hospital, and gave written informed consent after receiving oral and written explanations of the nature of the study. The ethics committee of Fujimoto General Hospital approved the experiments and confirmed that all experiments were performed in accordance with relevant guidelines and regulations.
Oddball Task
The standard auditory oddball paradigm was performed with a stimulus sequence in which the target tone was 2000 Hz with a probability of 20% and the non-target tone was 1000 Hz with a probability of 80%. Both stimuli were delivered binaurally by a sound stimulator for a duration of 100 ms, rise/fall time of 10 ms, an intensity of 80 dB SPL (sound pressure level), and a constant inter-stimulus interval of 2.3 s [33, 34]. Subjects were asked to silently count the number of target stimuli. Three sessions were recorded, each consisting of about 20 target stimuli. Short breaks were given to the subjects between sessions, but head movement was not allowed during breaks. All subjects detected more than 90% of the stimuli.
MEG Recording
MEG data were recorded in a magnetically shielded room at Fujimoto General Hospital using a 160-channel whole-head coaxial gradiometer MEG system (Yokogawa Electric Corporation, Kanazawa, Japan). The sensing coils in this system are 15.5 mm in diameter, with a 50 mm baseline and 23 mm separation between each pair of sensing coils. The subjects lay in the supine position with their eyes closed. MEG data were recorded through a 0.16-200 Hz band-pass filter with a sampling rate of 1000 Hz. In order to confirm P300 activities, we also recorded the EEG placed according to the international 10-20 system.
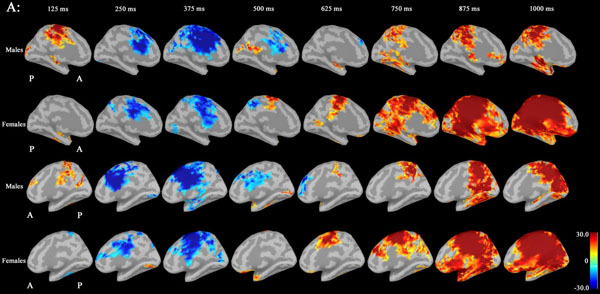
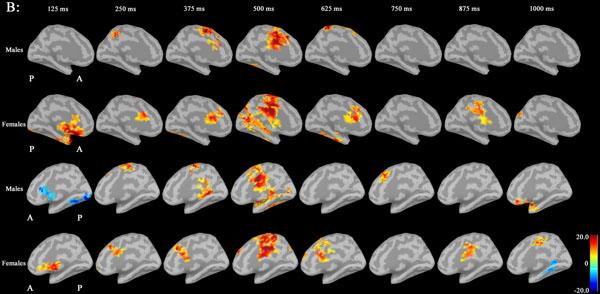
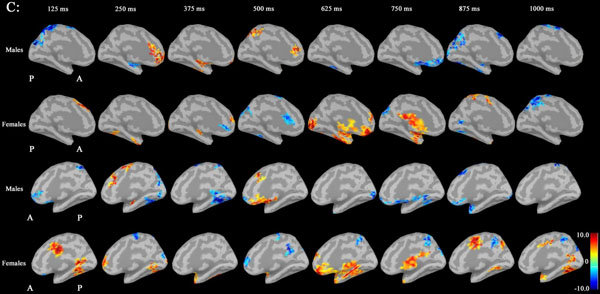
Data Analysis
Data were analyzed using Brainstorm 3.2 [35], a documented program that is available for free download online under the GNU general public license http://neuroimage.usc.edu/brainstorm.
Pre-processing
An artifact removal procedure using independent component analysis and based on visual inspection was applied to remove typical noise components (e.g., eye-blinks and heartbeats). An offline digital notch filter was applied to remove the power line interferences. Trials were epochal from -1000 ms before stimulus onset to 2000 ms after stimulus onset. Trials with unexpected, large artifacts were defined as bad trials and were excluded from further analyses. When we found a sensor with unexpected values that were not coherent with the other surrounding sensors, we defined it as a bad channel and excluded it from further analyses.
Source Estimation
From the subjects’ individual magnetic resonance image, we extracted the cortical surface by using FreeSurfer [36]. The brain cortical surface was downsampled to a total of 15,000 points in both hemispheres. The lead field (forward model of the brain) was calculated by the overlapping spheres method [37]. Source estimation was performed using the depth-weighted minimum L2 norm method [38]. The Desikan-Killiany atlas of FreeSurfer was adopted to apply anatomical labels.
Localization of Relative Changes
We were interested in the significant locations that were significantly related to the task in the gamma bands. Therefore, we localized them in source-space using the following procedure: 1) source time series were estimated using the source estimation described in the previous section. 2) The time series were filtered into the three bands: 30-50 Hz (low-gamma), 50-100 Hz (mid-gamma), and 100-150 Hz (high-gamma). 3) The amplitudes of these bands were obtained using the Hilbert transform. 4) Next, we averaged the amplitude in the time bands: the 50 ms-width window, 50 ms intervals and 50% overlap. 5) Then, the relative changes in the amplitude and in each voxel were calculated by dividing by the baseline time period (from -250 ms to -1 ms). 6) Finally, we obtained the cortical maps of relative changes in the amplitude of the specific gamma band. The units for these maps were percentage from the amplitude in the baseline period. To determine the tendency for the whole-brain level of the localization in the gamma bands, we calculated the grand average maps, which were averaged across males or females, and we calculated the statistical t test images between sexes.
Functional Connectivity Analysis
According to the significant sex differences in the statistical t test images of the localization of relative changes (Figs. 2 and 3), we chose significant three points which formed the peaks of the t-images. These three points belonged in the left posterior cingulate cortex (CGp), the right caudal anterior cingulate (cACC), and the left rostral anterior cingulate (rACC) regions of the atlas of Desikan-Killiany [39], respectively. We used these points as seed points and calculated between one of them and other cortical target regions.
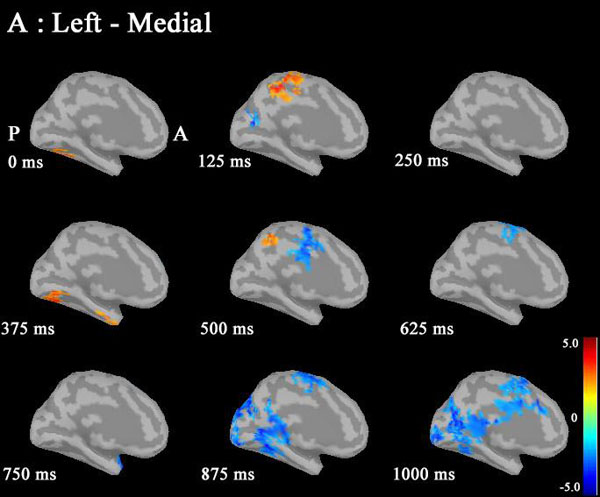
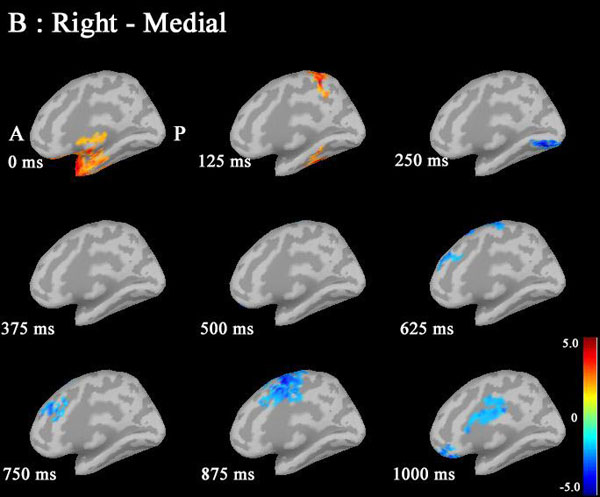
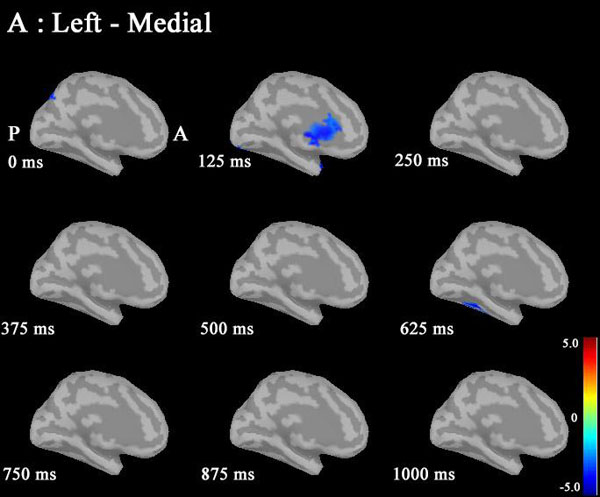
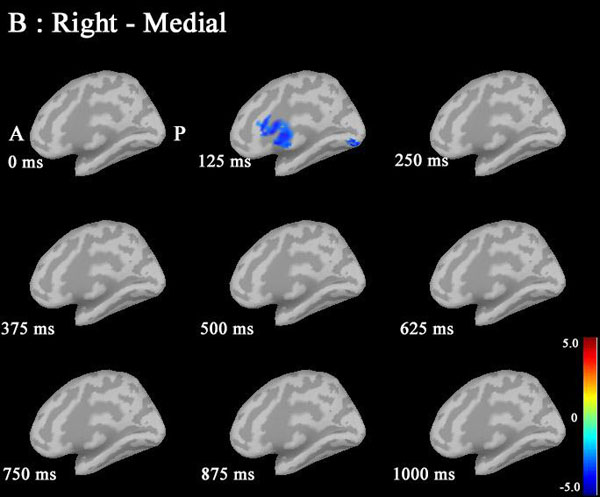
The corrected IC was adopted to estimate functional connectivity [31, 39-41]. The original imaginary coherence [42] can avoid spurious results caused by the algorithm leakage of source estimation. However, its intensity value was affected by the algorithm leakage. This problem was improved with a modified form of the IC whose intensity values were unaffected by the algorithm leakage. This corrected IC was denoted as
where ϕ is a complex coherence and
is the imaginary portion of the coherence, and
is the real portion of the coherence. Results of the IC analysis were then coordinated with significant differences in IC. Coherence between seeds and targets was computed for four time windows (0-250 ms, 250-500 ms, 500-750 ms, and 750 ms) and at three gamma bands (30-50 Hz, 50-100 Hz and 100-150 Hz). IC maps (source-coherence images) displayed sex differences as spatial distributions reflecting the degree of IC corresponding to the seed, that is, levels of strength in males compared to females were displayed on a color indicator (from red to blue, indicating strongest to weakest).
RESULTS
Noise was reduced by using Brainstorm 3.2. No significant differences between males and females in P300 amplitudes or latencies were shown with MEG or EEG (Table 1). Grand average maps of medial surfaces at the source level showed sex differences in ERD and ERS (Fig. 1). At 30-50Hz, ERS in males was increased in both CGp and paracentral regions, compared to females at 125 ms. ERD in males was increased in both cACC regions at 500 ms in both hemispheres. ERS in females was increased in both paracentral regions at 625 ms, compared to males (Fig. 1A). At 50-100 Hz, ERS in females was increased in the medial orbitofrontal regions at 125 ms, and cACC regions at 250 ms in both hemispheres (Fig. 1B). At 100-150Hz, ERS in males was increased in the left rACC region at 250 ms. ERD in females was increased in the left rACC region at 375 ms. ERS in females was increased in both medial temporal regions at 625 ms (Fig. 1B).Statistical source maps of differences in t values (p<0.05) showed sex differences. At 30-50 Hz (Fig. 2): in the sagittal image, ERS in males and was significantly increased in the paracentral region in both hemispheres at 125 ms (left > right), and in the right temporal region at 0 ms. ERD in males was increased in the left posterior cingulate cortex (CGp) at 500 ms. ERD was also increased in superior frontal regions in both hemispheres at 875ms, and in isthmus cingulate and lingual regions at 875ms and at 1000ms.
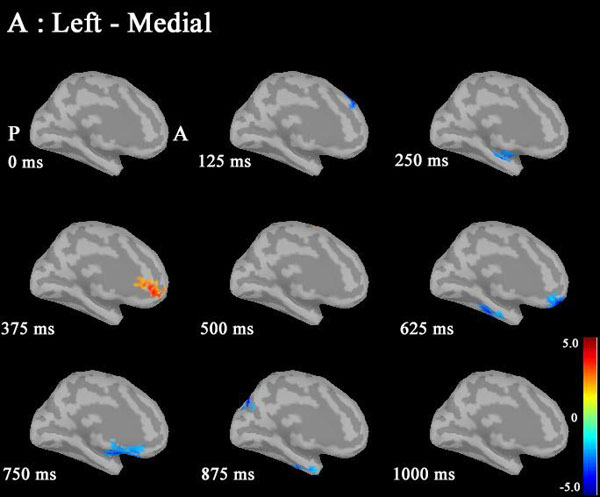
At 50-100 Hz: ERD in males was significantly increased in the caudal anterior cingulate cortex (cACC) regions in both hemisphers at 125 ms (Fig. 3A and B).
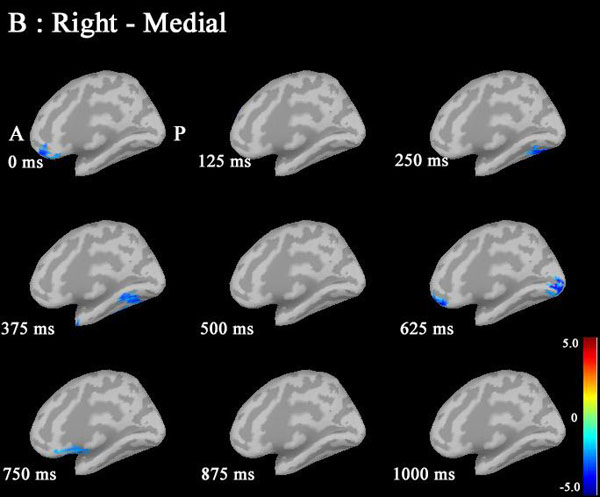
Mean | SD | p-value | ||
---|---|---|---|---|
Age(years) | Male | 27.89 | 4.00 | 0.30 |
Female | 25.99 | 5.72 | ||
Correct answer rate | Male | 0.97 | 0.02 | 0.76 |
Female | 0.97 | 0.02 | ||
P300 latency (ms) | Male | 333.80 | 24.64 | 0.45 |
Female | 325.00 | 36.86 | ||
EEG Amplitude (mV) | Male | -0.20 | 0.07 | 0.07 |
Female | -0.26 | 0.09 | ||
MEG Amplitude (fT) | Male | 269.40 | 88.33 | 0.35 |
Female | 239.99 | 80.15 |
At 100-150 Hz: ERS in males was significantly increased in the left rostral ACC (rACC) at 375 ms.
Based on the statistical source map of ERD/ERS, we chose three seed points that showed significant sex differences: left CGp (Motreal Neurological Institute (MNI) coordinates: X=-5, Y=-6, Z=32) at 500ms, left caudal ACC (cACC) (MNI coordinates: X=0, Y=19, Z=15) at 125ms, and right rACC (MNI coordinates: X=1, Y=41, Z=-3) at 375ms) (Fig. 4). Table 2 shows the ERD/ERS ratio and coordinate points for the three seed regions. We calculated IC between the seeds point and other cortical target regions.
Frequency | Time window | X | Y | Z | ERDERS ratio (%) | One sample t-test | Paired t-test | Region | ||||||
---|---|---|---|---|---|---|---|---|---|---|---|---|---|---|
Sex | Mean | 95% confidence interval | ||||||||||||
lower limit | upper limit | t-value | p-value | t-value | p-value | |||||||||
1 | 30-50Hz | 450-500ms | -5.0 | -6.0 | 32.0 | Male | -7.7 | -46.2 | 30.9 | -0.426 | 0.677 | -2.267 | 0.031 | Left-posterior cingulate cortex (CGp) |
Female | 41.4 | 14.8 | 67.9 | 3.323 | 0.005 | |||||||||
2 | 50-100Hz | 200-250ms | 0.0 | 19.0 | 15.0 | Male | 6.6 | -15.2 | 28.5 | 1.020 | 0.325 | -2.069 | 0.048 | Right-caudal anterior cingulate cortex (cACC) |
Female | 38.5 | 14.1 | 62.9 | 2.409 | 0.029 | |||||||||
3 | 100-150Hz | 325-375ms | 1.0 | 41.0 | -3.0 | Male | 28.4 | 11.2 | 45.6 | 3.549 | 0.003 | 2.289 | 0.030 | Left-rostral anterior cingulate cortex (rACC) |
Female | 1.3 | -17.1 | 19.8 | 0.152 | 0.882 |
In IC maps between the seed (left CGp; X=-5, Y=-6, Z=32) and targets at 30-50 Hz (Fig. 5), IC in males was increased in the following targets as follows: bilateral prefrontal, right inferior frontal and bilateral lateral occipital regions during 0-250 ms; bilateral frontal (left>right) regions during 250-500 ms; bilateral frontal (right>left) and bilateral medial parietal regions during 500-750 ms; and right hippocamapal and fusiform regions during 750-1000ms. IC in females was not increased in any regions.
In IC maps between the seed (right rACC; X=1, Y=41, Z=-3) and targets at 30-50 Hz (Fig. 6), IC in males was increased in the following targets as follows: the right superior parietal, bilateral orbitofrontal and ritht fusiform regions during 0-250 ms; bilateral frontal, bilateral superior parietal regions, right lateral orbitofrontal, right insula, right inferior temoporal, left precuneus, bilateral CGp and left insula regions during 250-500 ms; right frontal, left occipital, left inferior temporal and left fusiform regions during 500-750 ms; and right frontal, right inferior frontal and left occipital regions during 750-1000 ms. IC in females was increased in the supramarginal region during 500-750 ms.
In IC maps between the seed (left cACC; X=0, Y=19, Z=15) and targets at 30-50 Hz (Fig. 7), IC in males was increased in the following targets: the right superior parietal during 0-250 ms; bilateral frontal and bilateral superior parietal regions, right lateral orbitofrontal, right insula, right inferior temoporal, and left insula regions during 250-500 ms. IC in females was not increased in any regions. However, IC at 50-100 Hz and 100-150 Hz did not show common changes.
DISCUSSION
The source map of ERD/ERS in the gamma band showed sex differences in the distribution of the proportional spectral power, whereas the sizes and magnitudes of proportional power depended on specific time windows and the height of frequency during the oddball task. Sex differences in spectral power were noted in frontal and parietal regions, suggesting sex differences in information processing in these regions.
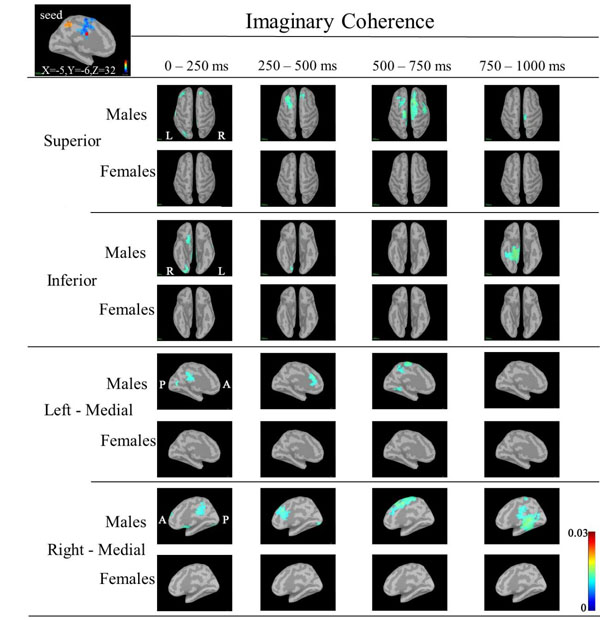
Statistical source maps of differences in t values (p<0.05) in the low gamma band (30-50 Hz) mainly showed a significant increase in ERD in males in the CGp at 500 ms. ERS in males was also increased in the paracentral region at 125 ms. (Fig. 2). ERS was also increased in the caudal ACC and the rACC in the mid-gamma bannd (50-100)Hz at 125 ms (Fig. 3). ERS in males was significantly increased in the rACC at 375 ms at 100-150 Hz (Fig. 4). The r ACC, c ACC (or midline cingulate cortex), and CGp including parietal regions were associated with sex differences in information processing.
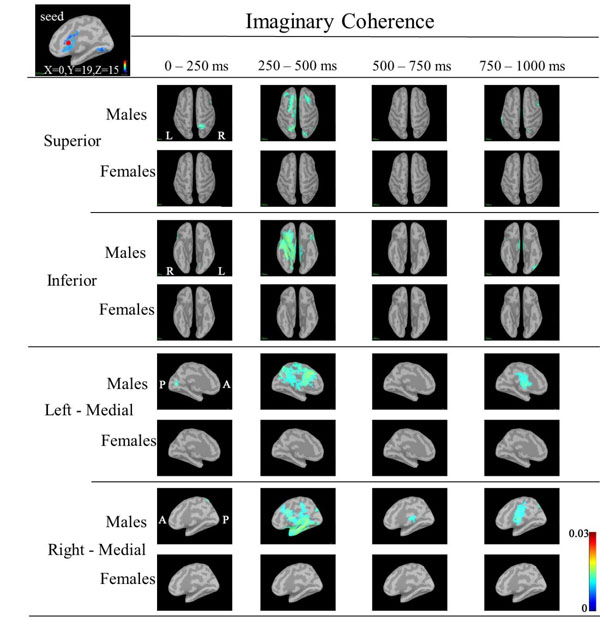
The ACC is primarily associated with generating of physiological or behavioral responses, and plays a regulatory role in these regions, such as in the top-down modulation of limbic and endocrine systems for the purpose of regulation of emotions [43]. The ACC is generally divided into the rACC and cACC and serves a variety of cognitive functions [42, 44].
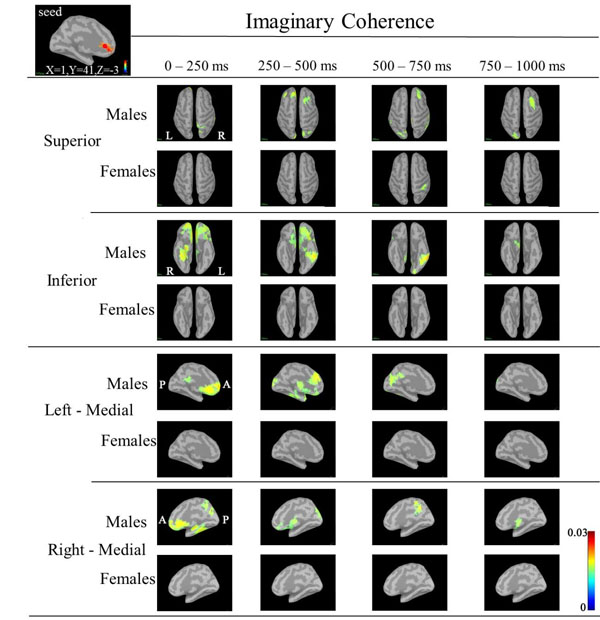
The rACC plays a regulatory role regarding the limbic regions that are involved in generating emotional responses [42] The finding that specral power in males was significantly increased in the rACC at 375 ms, reflected differences in emotional responses to the task. Males may grapple with the task more emotionally or aggressively than females.
The cACC or midcingulate cortex is hub where information about pain, threats, and other more abstract forms of potential punishment can be synthesized and used to modulate regions involved in expressing fear and anxiety, executing goal-directed behaviors, and biasing the focus of selective attention [44]. Significant decreases in spectral power in the cACC region at 125 ms in males may suggest sex differences in pain, threats and punishment involved in expressing fear and anxiety. Males may be relatively indifferent to fear and anxiety compared to females.
The spectral power in males was significantly decreased in the left CGp at the 500 ms time window in the low gamma band. CGp is a key node in the default node network and related to learning, memory, reward and task engagement [45]. CGp related to decision making [46]. Different parts of the cingulate cortex play essential roles in intuitive strategy decision-making, where as the CGp and ACC complementally encoded the defense and attack strategy values, respectively [47]. Decreased spectral power in males in the CGp region at 125 ms may be related to sex differences in intuitive strategies for decision making.
In addition, the spectral power in males was increased in the paracentral region at 125 ms. The parietal cortex is related to spatial analysis which depends on the integration of information from multiple regions, and cotributed episodic memory retrieval [48]. Changes in power in these regions may indicate that males process the task more spatially than females.
Based on the statistical source maps, we chose three regions (left CGp, right cACC and rACC) as seed regions in order to examine sex differences in IC between seed and other regions (taraget). We obtained IC maps of each of the three regions at low, mid and high gamma bands. IC maps at the low gamma band showed sex differences in IC, but IC maps at mid and high gamma bands did not show characteristic patterns of sex differences due to larger individuall differences. This is probably because sex differences in IC may be difficult to detect or may not existe at mid and high gamma bands. IC maps at the low gamma band corresponding to three seeds showed increased IC in target regions in males compared to females (Figs. 5-7).
Although IC in the low gamma band between the seed (left CGp or right cACC or rACC) and ceratin targets showed an increase in males, we noted that IC was markedly increased between the left rACC and a target such as the bilateral bilateral orbitofrontal or right fusiform regions during 0-250 ms, between the left rACC and bilateral frontal or bilateral orbitofrontal or left inferior temporal or left fusiform regions during 250-500 ms, between the left rACC and right frontal or left occipital or left inferior temporal regions during 500-750 ms, and between the left rACC and right frontal or left occipital regions during 750-1000 ms (Fig. 7).
These findings showed that the left rACC in males had close functional relationships with these target regions that dynamically changed with passage of processing time of the task. The left rACC had a functional relationship with frontal, orbitofrontal, inferior temporal and fusiform regions. Orbitofrontal cortex plays a pivotal role in valuation for incommensurable goods, a critical component process in human decision making [49]. Increased IC between the left rACC and orbitofrontal regions may reflect that males executed the task with value judgement. The increase in IC in males suggested that males may perform the task constructively, emotionally, and with analysis and that information processing in males was more complicated in the cortico-cortical circuit. On the other hand, females perfomed the task with general attention and economical well-balanced processing, resulting in little changes in IC. Economical information processing in females was explained by the higher overall cortical connectivity [50].
These sex differences in gamma bands may be related to differences in neurotransmitter systems and sex hormones in brain circuitry. Gamma oscillations emerge in early childhood, and maturation of neural synchrony is shown to be compatible with changes in myelination of cortico-cortical connections and with development of GABAergic neurotransmission [51, 52]. The GABAergic system plays an important role in the primary generation of high-frequency oscillations and local synchronization. As modulatory systems, GABAergic and glutamatergic connection dynamics play a key role as modulatory systems in the gating of oscillations and synchrony. GABAergic inhibition of local excitatory neurons and synchronous activity of fast-spiking interneurons generates gamma oscillations (30-80 Hz) [19, 53]. Inter-regional synchronization is shown to be the result of long-range inhibitory projections that originate from GABAergic cells and terminate selectively on inhibitory interneurons in the respective target areas. Such long-range inhibitory projections are present between the basal forebrain and the cortical mantle. A study of addiction showed that sex differences in dopaminergic function emerged during adolescence, and a hypothetical model of how estradiol and testosterone influenced the ontogeny of forebrain dopamine systems was proposed, including the hypothesis that GABAergic feedback on dopaminergic neurons may also contribute to additional regulation of dopaminergic functions in females [54]. One study of pediatric subjects showed progressive bilateral development of frontal P300 in females, whereas development of the frontal P300 amplitude in males is most prominent in the right hemisphere [55].
In addition, neuroanatomical sex differences are present in the ACC and corpus callosum [56-58]. Males have more pronounced leftward asymmetric doubling or gyri in the ACC than females, whereas females showed greater symmetry, with less fissurization of the left ACC [8, 59, 60], suggesting greater interhemispheric connectivity and possibly accounting for the more bilateral pattern of language-related activation in females [61]. In addition, females have shorter path lengths and decreased clustering coefficients in the delta band as compared to men, suggesting that the female brain has a more efficient network architecture [62]. A P300 study showed sex differences, which were explained by disparities in the degree or quality of interhemispheric interaction, related to the morphology of the corpus callosum and midcingulate folding asymmetry [8]. In our current study, females also responded more quickly to local targets, whereas men did not differentially respond to hierarchical stimuli in our event-related potential study. These results can be explained by biologically based differences in hemispheric asymmetry.
A limitation of our study is that we examined IC only in three seed areas, the left CGp, the right cACC and the rACC. If IC between all seeds and all other areas (such as parietal, temporal and occipital regions) showing differences in ERD/ERS was examined, other sex differences in IC may have been found; however, this would be a daunting task given the enormous number of possible connections between brain areas. Furthermore, not only connectivity between two areas, but among multiple areas, must be studied. In addition, our interpretation of the relationship between spectral power changes and the effects of personality structure is only speculation. Sex differences are complicated because they are related to not only to biological factors (such as genetic, physical, physiological, and hormonal factors) but also to psychological, social, cultural and intellectual factors. Further investigation is needed.
CONCLUSION
In conclusion, our study showed sex differences in ERD/ERS and IC in the gamma band during the classical auditory oddball task. IC between seeds (the left CGp or right cACC or the left rACC) and targets showed specific patterns of sex differences. Sex differences in regions, sizes and magnitudes of communication between neuronal groups were seen for specific time windows and the height of gamma bands. These findings were interpreted to indicate a more efficient network architecture in females, corresponding to higher overall cortical connectivity due to greater interhemispheric connectivity in the female brain. In addition, sex differences in ERD/ERS and IC in the gamma band may be related to sex hormones and neurotransmitter systems such as the GABAergic and dopaminergic systems. Measurement of IC using MEG was a useful method to study sex differences in functional brain connectivity.
CONFLICT OF INTEREST
The authors confirm that this article content has no conflict of interest.
ACKNOWLEDGEMENTS
Declared none.