All published articles of this journal are available on ScienceDirect.
Regional Parieto-occipital Hypoperfusion on Arterial Spin Labeling Associates with Major Depressive Disorder
Abstract
Background:
Reduced cerebral blood flow in parieto-occipital regions has been reported in neurodegenerative disorders using ASL. We aimed to investigate neuropsychiatric and neurodegenerative comorbidities that may associate with parieto-occipital region hypoperfusion.
Methods:
This was a retrospective single-center study. Between March 2017 to May 2018, adult patients who underwent brain MRI with the inclusion of ASL perfusion and who had bilateral reductions of CBF in the parieto-occipital regions were included. ASL was performed using a pseudo-continuous arterial spin labeling (pCASL) technique on 1.5T MR system. Age and gender-matched patients with no perfusion defect were concurrently collected. Comorbidity data was collected from EMR, including major depressive disorder, Alzheimer’s disease, Parkinson’s disease, Schizophrenia, anxiety disorder, hypertension, diabetes mellitus type II, coronary artery disease, and chronic kidney disease. A Pearson’s Chi-Square test was performed to assess for comorbidities associated with hypoperfusion of the parieto-occipital lobes.
Results:
Our patient cohort consisted of 93 patients with bilateral hypoperfusion in the parieto-occipital lobes and 93 age and gender-matched patients without corresponding perfusion defects based on ASL-CBF. Among the comorbidities assessed, there was a statistically significant association between hypoperfusion of the parieto-occipital lobes and major depressive disorder (p=0.004) and Parkinson’s disease (p=0.044). There was no statistically significant association for Alzheimer’s disease, generalized anxiety disorder, diabetes mellitus type II, hypertension, coronary artery disease, or chronic kidney disease.
Conclusion:
Major depressive disorder may be linked to regional parieto-occipital hypoperfusion on ASL.
1. INTRODUCTION
Arterial Spin Labeling (ASL) is a noninvasive MR perfusion method for quantifying Cerebral Blood Flow (CBF) by utilizing arterial water as a freely diffusible endogenous tracer [1-3]. These studies are easily reproducible and performed without gadolinium contrast. Among the ASL methods, the most commonly utilized is continuous ASL (CASL) and pulsed ASL (PASL). CASL induces continuous inversion of blood water spin as it passes a particular plane [4]. Conversely, PASL employs a single pulse to define a volume containing arterial blood for labeling. CASL has a greater signal-to-noise ratio, however, it is limited by imperfect practical implementation [5, 6]. Recently, pseudo-continuous ASL (pCASL), a hybrid ASL variant, is increasingly employed combining aspects of CASL and PASL by maintaining a high signal-to-noise ratio while being compatible with widely available body coils [5, 7, 8].
With increasing interest and applicability of ASL studies, there is a clinical interest in studying patterns of CBF as it relates to neuropsychiatric diseases. ASL studies for cerebral-occlusive disease [9-11], major depressive disorder (MDD) [12-16], Alzheimer’s disease (AD) [5, 17-19], chronic migraine [20], and Parkinson’s disease (PD) [21, 22] have been previously reported. Much of the earlier work in functional neuroimaging entailed measured baseline cerebral blood flow (CBF) and glucose metabolism using positron emission tomography (PET) and single-photon emission computer tomography (SPECT) [23, 24]. Resting-state fMRI studies subsequently followed examining the functional connectivity pattern among regions of the brain, however, there was a limitation when assessing objective biomarkers and specific regions of abnormal flow [25].
Given the considerable success and utility of these prior modalities in neurodegenerative populations and relative ease of obtaining ASL in conjunction with conventional MR sequences, ASL has been under investigation for similar purposes. A growing number of ASL studies have emerged in recent years, assessing its utility in assessing neurodegenerative disorders that have been reported in prior ASL studies. For example, studies in patients with PD report hypoperfusion in the parieto-occipital region [22, 26-28]. ASL studies in patients with AD have reported hypoperfusion in the parieto-occipital [20, 22] and frontotemporal region [17, 19]. However, neuropsychiatric disorders associated with CBF abnormality have not been studied extensively in prior ASL studies. Current literature show variable results in MDD [24, 29] with CBF abnormality in the prefrontal cortex [13, 14, 30, 31], anterior cingulate cortex [14, 30, 31], subgenual cingulate [14], basal ganglia [30, 32], and superior insula [30] with variability in CBF perfusion as well as asymmetry [12].
In recent years, there has been increasing interest and understanding of CBF abnormalities on ASL in association with neurodegenerative disease. We have noticed a subset of patients with regional hypoperfusion, specifically in the parieto-occipital lobes. In this study, we investigated a potential link between regional parieto-occipital hypoperfusion and underlying common medical and neurodegenerative/ psychiatric disease.
2. MATERIALS AND METHODS
2.1. Study Population
This is a single-center retrospective study, which was approved by the local Institutional Review Board with a waiver of informed consent. From March 2017 to May 2018, a total of 1026 consecutive patients who presented to our inpatient and emergency department underwent brain MRI with the inclusion of ASL (Fig. 1). One hundred and ten of these patients had regional parieto-occipital hypoperfusion (Fig. 2). We excluded patients with any perfusion defect outside of the parieto-occipital region.
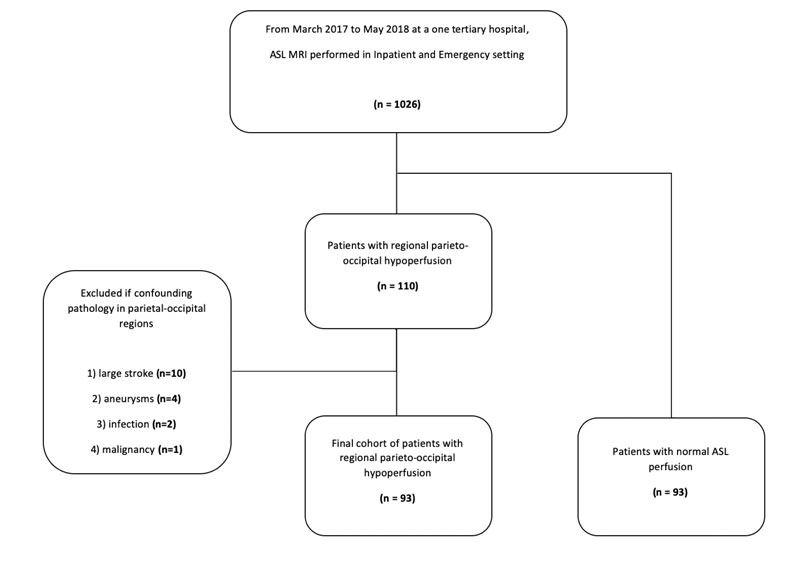
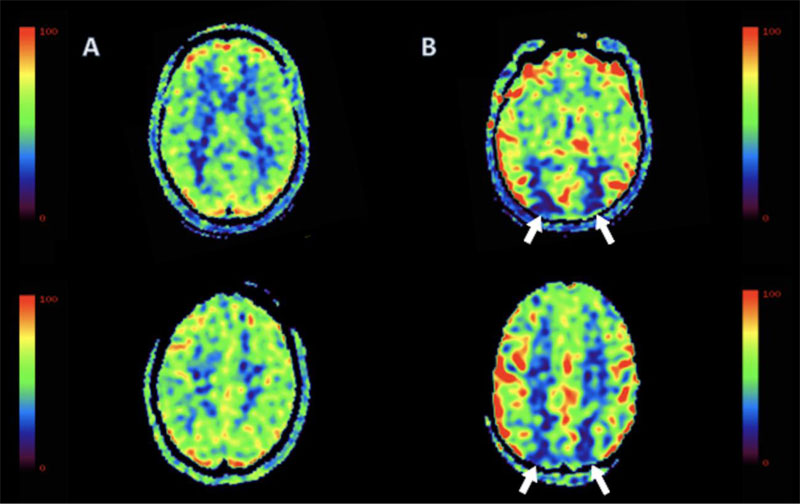
We further excluded patients with CVA (n=10), aneurysm (n= 4), infection (n=2), and malignancy (n=1) in the parieto-occipital region. Our final cohort included 93 patients. Age and gender-matched patients with no corresponding perfusion defect were concurrently collected from the same database. Comorbidity data was collected from our EMR system, including MDD, Generalized Anxiety disorder, Alzheimer’s dementia, PD, Schizophrenia, hypertension, diabetes mellitus type II, coronary artery disease, and chronic kidney disease. Comorbidities were diagnosed by the primary or psychiatric team and retrospectively obtained from chart review. Neuropsychiatric conditions such as MDD, generalized anxiety disorder, and Schizophrenia were made per the Diagnostic and Statistical Manual of Mental Disorders (DSM-5). Data on cataract history, smoking history, and prior history of non-parietal-occipital strokes were also collected.
2.2. Image Acquisition and Image Analysis
Imaging protocol for brain MRI included the acquisition of sagittal T1, axial T2, axial GRE, axial FLAIR, axial DWI, and axial ASL. Image acquisition was performed using a 1.5-T clinical MR imaging unit (GE Optima MR450w; GE Medical Systems, Milwaukee) with an eight-channel head coil for signal reception. ASL was performed using a three-dimensional pseudo-continuous ASL (pCASL) with a fast spin-echo stack-of-spiral readout with eight interleaves. Each spiral arm included 512 sampling points. The following parameters were applied: TR/TE 4525/11 ms, FOV: 24 x 24 cm, matrix size: 64 x 64, 30 slices each 4 mm thick, and NEX = 3. Pseudo-continuous spin labeling was performed for 1.5 seconds before a post–spin-labeling delay of 2 seconds. Background suppression was achieved by applying saturation pulses inferior to the labeling plane, allowing for the increase in the sharpness of the bolus [33]. This setting resulted in the acquisition of 3D voxel size of 3.8 × 3.8 × 4 mm3 acquired in 4 minutes and 30 seconds.
Image reconstruction was performed online by the scanner software. Pairwise subtraction between label and control images was obtained and averaged to generate the mean difference and converted to CBF maps based on a previously published model [34]. After automated reconstruction by the scanner software, DICOM CBF maps along with all other anatomical imaging, were sent to PACS and available for interpretation.
One board-certified neuroradiologist reviewed the ASL-CBF MR images on PACS (Centricity, GE Healthcare, Chicago, IL). The observer was blinded to the radiological reports. If there was a subjective reduction in CBF in the parieto-occipital lobes, then the case was interpreted as hypoperfusion and included in this study.
3. RESULTS
3.1. Clinical Characteristics of Patient Population
Our patient cohorts consisted of 93 patients with bilateral hypoperfusion in the parieto-occipital lobes and 93 patients with no perfusion defect based on ASL-CBF. Demographic comparison of the two cohorts revealed no statistical difference in gender, age, or history of prior strokes (Table 1). The mean ± standard deviation of age (years) was 75 ± 13, with a median of 76 for the cohort with occipital hypoperfusion and 75 ± 11 with a median of 76 for the normal control.
Category | Normal CBF control (N=93) |
rCBF hypoperfusion (N=93) |
P value |
---|---|---|---|
Demographic Age (mean ± SD; median) |
75 ± 11; 76 | 75 ± 13; 76 | 0.87 |
Gender (M/F) | 61/32 | 61/32 | 1 |
Diabetes Mellitus type II | 39 | 40 | 0.88 |
Hypertension | 71 | 69 | 0.73 |
Chronic Heart Failure | 14 | 12 | 0.67 |
Coronary artery disease Chronic kidney disease History of malignancy History of CVA History of smoking |
36 27 31 26 19 |
27 20 20 34 19 |
0.16 0.24 0.07 0.21 1 |
Neuropsychiatric Major Depressive Disorder |
13 | 29 | 0.004* |
Generalized Anxiety Disorder Schizophrenia Neurodegenerative Alzheimer’s Dementia |
4 2 11 |
9 2 18 |
0.15 1 0.16 |
Parkinson’s disease | 3 | 10 | 0.04* |
*P<0.05 on Pearson’s Chi Square test.
3.2. Association of MDD and PD to Hypoperfusion of Parieto-Occipital Lobe
Among the comorbidities assessed, there was a statistically significant association between hypoperfusion of the parieto-occipital lobes with MDD (p= 0.004) and with PD (p= 0.04) (Table 1). Compared to 13 patients in the aged-matched cohort, there were 29 patients with MDD in the cohort with parieto-occipital hypoperfusion. In our analysis of PD, there were 10 patients with PD compared to 3 in the aged-matched cohort. Of note, there were more patients with Alzheimer’s disease in our cohort with parieto-occipital hypoperfusion (n= 18) compared to aged-matched control (n= 11), but the difference did not reach statistical significance (p= 0.16).
No significant associations were found for Schizophrenia (p= 1.0) or anxiety disorder (p= 0.15). Common inpatient comorbidities such as diabetes mellitus II (p= 0.88), hypertension (p= 0.73), coronary artery disease (p= 0.16), chronic kidney disease (p= 0.24), or malignancy (p= 0.07) did not yield a statistically significant difference.
4. DISCUSSION
Perfusion abnormalities in the parieto-occipital region have been extensively studied in prior ASL studies, especially in neurodegenerative disorders such as Parkinson’s and Alzheimer’s disease. In our single-center institution, we found a significant portion of our patients with bilateral parieto-occipital hypoperfusion as an incidental finding. To understand this CBF abnormality further, we assessed for neuropsychiatric and other neurodegenerative disorders that may associate with this perfusion defect by comparing this cohort to age and gender-matched cohort without corresponding perfusion defects.
In our study, we found that MDD and PD associate with bilateral parieto-occipital hypoperfusion. CBF abnormalities in the prefrontal cortex [13, 14, 30-32, 35] and anterior cingulate cortex [14, 30-32] have been well documented in the past in these pathologies. To our knowledge, MDD association with bilateral parieto-occipital hypoperfusion has not been described before.
The parieto-occipital cortices have numerous anatomical interconnections with cortical and subcortical pathways governing attention, spatial representation, working memory, eye movements, and guidance of action [36, 37]. Furthermore, parietal lobe dysfunction has been proposed to associate with depression [38], where they found that tactile learning problems to assess parietal lobe dysfunction was significantly impaired in patients with MDD, and rCBF using the xenon 133 inhalation technique was significantly decreased in the temporo-parietal lobes in patients with MDD [39]. Interestingly, Alosco et al. [40] using a Transcranial Doppler (TCD) showed a decrease in CBF in the bilateral MCA associating with significant depressive symptoms in patients with CHF. Their findings suggest a possibility that hypoperfusion of the parietal and occipital cortex may be contributing to the symptoms of depression in these patients. It has been proposed that flow reductions in these regions may correspond to impairment of temporo-parietal polymodal association area, which has strong reciprocal connectivity with prefrontal polymodal association cortex, which governs arousal, attentional, and motivational functions that are impaired in MD [36, 37]. Further analysis of the chronology of onset and progression of depression in a prospective study may be able to shed light on whether this CBF pattern may have predictive value or may correlate with disease progression.
The association of parieto-occipital CBF hypoperfusion is well documented in PD in prior ASL studies [22, 26-28] and observed in our current study. While frontal dysfunction is the prominent feature in PD, a posterior pattern of CBF abnormality affecting the posterior cingulate, precuneus, and occipital regions is known [26]. Furthermore, CBF perfusion reductions in posterior regions have been associated with cognitive decline in PD [28, 41]. Firbank et al. [28] demonstrated that the degree of parieto-occipital lobe CBF reduction associates with cognitive assessment score (CAMCOG) in patients with PD with dementia. Furthermore, parieto-occipital CBF reduction is likely linked to developing dementia in these patients with PD. These lobes are involved in visual-spatial processing, a function that is well documented to be abnormal in PD [42-44].
Prior ASL studies in patients with Alzheimer’s dementia have reported hypoperfusion in the parieto-occipital lobes [20, 22, 45]. Interestingly, although there were more patients with AD in our cohort with posterior hypoperfusion (n=18) compared to age-matched control (n=11), there was no statistically significant difference. Frontal and lateral temporal hypoperfusion has been documented in AD in prior studies [17, 19, 46]. As our cohort consisted exclusively of bilateral parieto-occipital hypoperfusion, there were likely cases with frontal or temporal hypoperfusion that were excluded from analysis, thus contributing to the lack of significant difference in our cohort. Additionally, prior studies have shown that CBF may be affected by several medications, including cholinesterase inhibitors, with treatment response correlating to an increase in perfusion and a relative return to baseline CBF in the frontotemporal and parieto-occipital region [47, 48]. A difference in cohorts may not be observed depending on the treatment status of these patients.
There are several limitations to our study. First, it was a retrospective study. A prospective study would have allowed us to conduct serial ASL studies to assess CBF pattern as it relates to the onset and progression of the disease. It would also allow us to structure the study design to reduce confounders, for example, including only patients with MDD without neurodegenerative disease and focusing on a single pathology at a time. Future studies may focus on prospectively recruiting patients with MDD without any other comorbidities and assessing for regional hypoperfusion. Secondly, having an objective scoring system for assessing the severity of depression, such as a Montreal Cognitive assessment or Hamilton depression scale, would have enhanced our study. Unfortunately, these were not documented in our patients. We also acknowledge that diagnosis of the assessed comorbidities, especially the underlying neuropsychiatric conditions, may vary across the diagnostic approach and specialty of the clinician.
Another limitation was that our MDD patients were treated at the time of imaging, and different SSRI/SNRI/Atypical antidepressants may have altered CBF pattern as was described in AD studies with cholinesterase inhibitors [47, 48]. Lastly, we acknowledge that this is a single-center study and our cohort consisted of patients from an inpatient and emergency setting obtaining ASL MR imaging for a variety of neurological disorders, which is not representative of the general population.
CONCLUSION
The major depressive disorder may be linked to regional parieto-occipital hypoperfusion on ASL. Future studies can focus on the evaluation of this association with other MR perfusion or CT perfusion imaging as well as assessing CBF pattern variation with onset and progression of MDD.
LIST OF ABBREVIATIONS
ADL | = Alzheimer’s Dementia |
ASL | = Arterial Spin Labeling |
MDD | = Major Depressive Disorder |
PD | = Parkinson’s Disease |
pCASL | = Pseudo-continuous Arterial Spin Labeling |
AUTHORS' CONTRIBUTIONS
Study concept and design, collection of data, analysis and interpretation of data, writing of manuscript, critical revision of manuscript. CL: collection of data. KM: Study concept and design, writing of manuscript, critical revision of manuscript. PB: Study concept and design, writing of manuscript critical revision of manuscript. All authors read and approved the final manuscript.
ETHICS APPROVAL AND CONSENT TO PARTICIPATE
This study was approved by the Mount Sinai Hospital Institutional Review Board under consent no (IRB# MS312-789).
HUMAN AND ANIMAL RIGHTS
Not applicable.
CONSENT FOR PUBLICATION
A waiver of informed consent was obtained from the Mount Sinai Hospital Institutional Review Board as this was a retrospective study.
AVAILABILITY OF DATA AND MATERIALS
The authors confirm that the data supporting the findings of this study are available within the article.
FUNDING
None.
CONFLICT OF INTEREST
The authors declare that they have no conflicts of interest.
ACKNOWLEDGEMENTS
Declared none.